His Fraudulency
Joe Biteme, properly styled His Fraudulency, continues to infest the White House, we haven’t heard much from the person who should have been declared the victor, and hopium is still being dispensed even as our military appears to have joined the political establishment in knuckling under to the fraud.
One can hope that all is not as it seems.
I’d love to feast on that crow.
Justice Must Be Done.
The prior election must be acknowledged as fraudulent, and steps must be taken to prosecute the fraudsters and restore integrity to the system.
Nothing else matters at this point. Talking about trying again in 2022 or 2024 is hopeless otherwise. Which is not to say one must never talk about this, but rather that one must account for this in ones planning; if fixing the fraud is not part of the plan, you have no plan.
Lawyer Appeasement Section
OK now for the fine print.
This is the WQTH Daily Thread. You know the drill. There’s no Poltical correctness, but civility is a requirement. There are Important Guidelines, here, with an addendum on 20191110.
We have a new board – called The U Tree – where people can take each other to the woodshed without fear of censorship or moderation.
And remember Wheatie’s Rules:
1. No food fights
2. No running with scissors.
3. If you bring snacks, bring enough for everyone.
4. Zeroth rule of gun safety: Don’t let the government get your guns.
5. Rule one of gun safety: The gun is always loaded.
5a. If you actually want the gun to be loaded, like because you’re checking out a bump in the night, then it’s empty.
6. Rule two of gun safety: Never point the gun at anything you’re not willing to destroy.
7. Rule three: Keep your finger off the trigger until ready to fire.
8. Rule the fourth: Be sure of your target and what is behind it.
(Hmm a few extras seem to have crept in.)
Spot Prices.
Kitco Ask. Last week:
Gold $1893
Silver $27.91
Platinum $1172
Palladium $2890
Rhodium $21,000
This week, markets closed as of 3PM MT.
Gold $1877.40
Silver $28.02
Platinum $1153.00
Palladium $2854.00
Rhodium $22,000.00
Gold was actually just below $1900 at open. The others have changed even less on a percentage basis. Since rhodium didn’t just jump right back up to nearly $30K, I’m thinking this price might not be a short term “spike” (but downward).
(Be advised that if you want to go buy some gold, you will have to pay at least $200 over these spot prices. They represent “paper” gold, not “physical” gold, a lump you can hold in your hand. Incidentally, if you do have a lump of some size, doesn’t it give you a nice warm feeling to heft it?)
The Atom
(Part VII of a Long Series)
Introduction
The general outline of this story is to start off by putting you “in touch” with the state of physics at the beginning of 1895. Physicists were feeling pretty confident that they understood most everything. Sure there were a few loose ends, but they were just loose ends.
1895 marks the year when people began tugging at the loose ends and things unraveled a bit. In the next three years, three major discoveries made it plain there was still a lot to learn at the fundamental level.
Once I’m there I will concentrate on a very, very small object…that ties in with stars, arguably the biggest objects there are (galaxies are basically collections of stars). And we would never have seen this but for those discoveries in the 1890s.
It’s such a long story I decided to break it down into pieces, and this is the seventh of those pieces. (Though to be sure this series seems to have taken on a life of its own.)
And here is the caveat: I will be explaining, at first, what the scientific consensus was in 1895. So much of what I have to say is out of date, and I know it…but going past it would be a spoiler. So I’d appreciate not being “corrected” in the comments when I say things like “mass is conserved.” I know that that isn’t considered true any more, but the point is in 1895 we didn’t know that. I will get there in due time. (On the other hand, if I do misrepresent the state of understanding as it was in 1895, I do want to know it.)
Also, to avoid getting bogged down in Spockian numbers specified to nine decimal places, I’m going to round a lot of things off. I used 9.8 kg m/s2 in Part for a number that’s actually closer to 9.80665, for instance, similarly for the number 32. In fact, I’ll be rounding off a lot today.
NOTE: A YUUUGE debt is owed here to “Discovery of the Elements,” 2nd edition, by James L. Marshall.
Why Talk About Atoms?
This post is going to seem like it actually is about chemistry, and in many ways it is.
However, physics and chemistry are right next door to each other. Physics is the most fundamental of the sciences, the others build on it, with chemistry being the one directly “on top” of the physics foundation. Thus it’s the major branch of science most directly connected to physics. And you’ll see some of that here. (Of course, where we divide sciences into major branches is largely arbitrary. For example, if there were a major branch for electricity and magnetism, it’d be tied even closer to physics than chemistry is, but in fact, E&M is considered a branch of physics rather than a major science in its own right.)
Phlogiston
Our story begins with Georg Ernest Stahl, in the 1600s. Before he came on the scene, what we now think of as chemistry was still under the sway of the alchemists, many of whom were trying to turn lead (and other base metals) into gold.
They had a basic theory of chemistry, to wit that the world was made of exactly four basic substances, earth, water, air, and fire (and in some cases they believed the heavens were made out of “aether”, something not encountered “down here”). Everything we see around us, they maintained, was some sort of mixture of these basic elements. So to change lead into gold, all one needed to do was change the mixture, removing some things and adding others.
Of course, that never came to anything, but during all their efforts they amassed a huge amount of knowledge about what would happen if you mixed certain things together and treated them in certain ways.
For example you could mix potash and sulphur, and create liver of sulphur. But you could also create liver of sulphur by heating vitriolated tartar together with charcoal.
(I use the older names here so that you can see how totally arbitrary this must have seemed to the people who used those names.)
So what we had by the 1600s was a vast collection of information like this, with no real way to connect the pieces and understand what was really going on.
And this is where Georg Ernest Stahl comes in.
He was the first to put forward a theory that seemed to tie this disparate trivia together. The theory could also be used to make predictions about what would happen with previoiusly untried processes. This would help tremendously if the theory were right, but would also, if the theory were wrong, allow it to be discredited because it had made a specific prediction that hadn’t come to pass.
(A lot of things people believe are “unfalsifiable.” That means there’s no way, even in principle to disprove it even if it’s wrong. Most real “conspiracy theories” are like this, actually; if any evidence is turned up against the theory, the advocates will dismiss it as falsified as part of the cover up. If your hypothesis can explain away anything this way, and you can disregard evidence against your hypothesis, you can’t be convinced it’s wrong, and the theory itself is worthless since it can be neither proved or disproved, and can make no meaningful predictions, either–any outcome can be made to fit the theory, so any outcome is possible if the theory is true.)
So here it is: Stahl noted some similarities between combustion (burning things), calcining (rust, corrosion), and respiration (both plant and animal “breathing”). He concluded that at a very basic level three of these four were the same thing–he excepted plant respiration, but claimed that it was fundamentally animal respiration in reverse.
His proposed explanation for all of these processes? That wood, when it burns, and metal when it rusts, and animals when they breathe, all give off a substance called phlogiston. Thus, the calx of some metal, say iron rust was a purer substance than the metal, because the metal had given up phlogiston to turn into the rust. Similarly, when you burned a log you could even hear it hiss as the plogiston was released.
The ancients had believed that fire, once released, went up into the heavens; Stalh believed that plogiston combined with the atmosphere, to form phlogisticated air.
Plants would simply recapture the phlogiston from the air, turning the air into dephlogisticated air, and incorporate it into their tissues, forming a sort of closed cycle, ready to be burned again, or eaten by an animal that would breathe and release the phlogiston into the atmosphere once again.
OK, there were a couple of simple objections to this. Wood, when burned would lose weight, but metals, when rusting, gained weight. But it was readily noted that burning wood released a lot of smoke, which surely weighed something, and it was presumed there was a weight gain there, too (which in fact is the case). It was proposed, therefore, that phlogiston had negative weight. This was a concrete prediction of the theory, that phlogiston, if isolated, would have what amounted to antigravity, or as they called it back then, levity.
Chemists made a bunch of progress in the 1770s towards proving this theory and bringing some order to chemistry, much like Newton had done with mechanics a century earlier.
Unfortunately things fell apart under the weight of too much evidence. Too much special pleading had to occur to explain away anomalies.
Phlogisticated air was produced by Daniel Rutherford in 1772. He would burn a candle in a closed container, let a mouse asphyxiate (which took about 15 minutes) in a closed container, and also could get metal to calcine in a closed container. The resultant gas from one of these processes (say the burning candle) could be tested in another (the mouse) and fail to support the new process as well, which gave him a warm fuzzy that all three gases were actually the same thing; air loaded to capacity with phlogiston.
Dephlogisticated air was prepareed by Scheele and Priestly separately but almost simultaneously in 1774. Scheele heated calx of mercury and collected the gas that came out; that gas would support combustion and respiration quite nicely so clearly it was air with no phlogiston in it at all.
Phlogiston was isolated by Henry Cavendish. This is the same Henry Cavendish who determined the value of the gravitational constant G over in physics land, as described in Part I of this series.
Cavendish added Mars (iron) to oil of vitriol to produce a gas which he collected in a bladder. The bladder actually floated in the air, which meant that he likely had phlogiston (which was supposed to have negative weight, after all), and the gas was also very combustible; logical for something released during burning. This new gas was “inflammable air” and had also been identified as being phlogiston.
So this looked very good for Stahl’s theory! Equations consistent with it could be written and phlogiston had indeed turned out to have negative weight.
We could even demonstrate that sulphur was oil of vitriol mixed with phlogiston, by use of those first two reactions I mentioned at the very beginning of this story.
By looking at all of this, it was clear that metals were compounds, and so was sulphur. The calxes and oil of vitriol were most likely pure substances, elements, irreducible to anything more simple.
Along comes Anoine Lavoisier. He made a fairly obvious prediction. Reacting phlogiston/inflammable air with dephlogisticated air should produce phlogisticated air.
It was already known that inflammable air was quite combustible, so Lavoisier built a very sturdy chamber for the reaction, one that would withstand the stress of the kaboom! and retain the product.
So then he did it, using a spark to touch off the reaction, and on examining the result he did not find phlogisticated air. Instead, he found the element water. And nothing else!
Think about that. There were other reactions that produced elements. But they always also produced something else. Starting with zinc and de-phlogisticated air, you could get the zinc calx element, but phlogisticated air would also be produced. In other words, if you start with a non element and turn it into an element, part of the original compound has to go somewhere else.
(zinc calx + phlogiston) + dephlogisticated air ->
zinc calx + phlogisticated air.
You can’t start with those sorts of beginning ingredient and end up with only an element afterwars. Whatever you broke away from the element has to have gone somewhere, in this case into the air to phlogisticate it.
So what’s going on here? How do you combine things and only get an element?
Fortunately, Lavoisier was a genius, and he did figure it out. By overturning every assumption that had been made.
He figured that water was a compound, a compound of inflammable air and dephlogisticated air. Up until this point water was presumed to be an element.
And that there was no such thing as phlogiston, and everything understood up to then was backwards.
If you understand modern chemistry at all, everything I’ve described up until now should seem inverted, like phlogiston is filling the role of oxygen, but in reverse–it is leaving things as they burn or rust, instead of combining with them.
But now, thanks to Lavoisier, try the new words “oxygen” for “dephlogisticated air” and “hydrogen” for “phlogiston” and “nitrogen” for “phlogisticated air.” These, Lavoisier realized are all elements; and air was a mixture of nitrogen and oxygen.
The metals weren’t compounds of something plus a “calx,” rather the calx was a compound of the metal and oxygen. And oil of vitriol was a compound of sulphur, not the other way around. (In fact today, oil of vitriol is called “sulphuric acid,” suitable for imbibing by your favorite Deep Stater.)
After several years of effort, Lavoisier was able to correctly identify 31 substances as elements, two still bear the names he gave to them (hydrogen and oxygen). Seven of these elements had not been isolated yet, but he figured they were part of a known compound; those are chlorine, fluorine, boron, calcium, magnesium, barium, and silicon.
Oddly he didn’t realize that potash and soda were similar; he thought they were compounds of ammonium. And he thought that heat and light were elements. (This was corrected by Count Rumford, who married Lavoisier’s widow.)
All in all, mistakes aside, this is a staggering amount of insight.
But he went further. In collaboration with three other chemists, he devised the naming system we use today. “Sodium chloride” is named according to this system; it indicates a compound of the two elements, sodium and chlorine. Gone was “flowers of zinc” to be replaced by “zinc oxide.” “Liver of sulphur” was now “potassium sulfide.” “Corrosive icy oil of tin” is now “stannic chloride.” And on and on, the new names reflecting the actual elemental composition. Most of the old names are now forgotten, but every once in a while you still hear them.
And now that elements were correctly identified, a lot of real progress could be made, because the whole mental map of what was going on was no longer upside-down and inside-out.
This is why Lavoisier is called “the Father of Chemistry.”
He was also a tax collector for Louis XVI. This made him well versed in accounting, which showed in his meticulous measuring of the masses of everything in reactions, to make sure the books balanced. He had demonstrated that mass was conserved in all chemical reactions.
Unfortunately his day job put his head into the guillotine in 1792 during the French Revolution. As Comte de Joseph-Louis Lagrange put it, “It required but a moment to cut off his head and perhaps a hundred years will not suffice to produce another like it.”
It took a long time for Lavoisier’s new chemistry to be accepted in Germany (the homeland of Stahl) and the United Kingdom was resistant as well. Politics had some influence on science back then too. But in England, it didn’t take too long. Because John Dalton would soon be hard at work, and so would Humphry Davy. These two parts happen almost simultaneously.
John Dalton
John Dalton made measurements of the masses of all reactants in many different reactions and came to the realization that elements reacted in certain fixed proportions by mass. (He managed this in spite of not being nearly as proficient at measurement as Lavoisier had been.) For example one unit of hydrogen appeared to react with 5.66 units of oxygen to form water. On the basis of this, he speculated that elements consisted of small minimum units, which he named atoms from Greek atomos, “can’t be cut.” This revived a speculation than had been dormant for over two thousand years, since Democritus who lived roughly around 400 BCE. He began publishing his work in 1806.
Dalton determined, very roughly, a lot of these ratios, and the ratios became what today are called “relative atomic masses.” The are the masses of atoms, relative to some (back then) unknown reference value. (In casual speech they are “atomic weights” and sometimes “atomic masses” though the latter can be confused with the actual mass of an atom in kilograms. Both “relative atomic mass” and “atomic weight” are officially sanctioned terms, though “atomic weight” seems to be falling out of favor. After all weight is actually a misnomer.)
Dalton carefully refined his table of atomic weights, but even his last effort is barely recognizable today. He had finally measured the oxygen:hydrogen ratio as 7, which was still not right, even given some of the bad assumptions he was making.
A lot of very basic (to us today) concepts were missing from this endeavour. It wasn’t clear that hydrogen and oxygen are never present as single atoms, but rather they’d form a compound with themselves, two hydrogen (or oxygen) atoms pairing off as a molecule of H2 or O2. Compounded atoms got the name “molecule.” This was true of nitrogen as well.
(On the subject of these diatomic elements, my high school chemistry teacher used to say that those elements whose names end in G, E, N or I, N, E were the “fags of the chemical world” because they’d form molecules with themselves. H2, O2, N2, F2, Cl2, Br2, I2 [for hydrogen, oxygen, nitrogen, fluorine, chlorine, bromine, and iodine, respectively]. I can guarantee you no high school teacher says that today. In any case, hydrogen has one bond, and shares it with the other hydrogen atom, oxygen has two bonds, and so is double bonded to the other oxygen atom in the molecule, nitrogen has three and triple-bonds. The “-ine” elements are all one bond each and are called, collectively, halogens.)
Also missing was the concept of valence; Dalton didn’t realize that it was possible for one atom to combine with more than one other atom, or even two or three times to the same other atom, and that different elements followed different rules in regards to this. Thus he never understood that water was H2O, not just HO. That caused him to understate oxygen’s atomic weight by a factor of two. He should have got oxygen = 8 on the basis of this misunderstanding, but he never quite got there.
All this emphasis I place on what he did not understand might lead you to think I am dumping on Dalton. No, absolutely not! Even with the things he didn’t know, he had made a huge conceptual leap, which (not incidentally) was needed before we could learn more. Ironically, the things he got right eventually made it possible for us to see his mistakes.
Amadeo Avogadro
Dalton’s misunderstanding of valence was corrected in part due to Amadeo Avogadro, who noted that when working with gases, their volume appeared to match these ratios. For instance a certain volume of hydrogen weighed two grams, matching its molecular weight; the same volume of oxygen would weigh 32 grams, matching O2‘s molecular weight. And when reacting, some volume of oxygen would combine with twice that volume of hydrogen to form water, in accordance with the H2O molecular formula for water, and not leave anything left over. Avogadro showed that at a given temperature and pressure, a certain volume of gas would contain the same number of molecules, regardless of which gas it was. Hydrogen, oxygen, Eric Swalwell’s most recent meal, it was all the same number of molecules per liter.
Today we know that 22.4 liters of gas at standard temperature (25 C) and pressure (1 atmosphere) will weigh, in grams, its molecular weight. That much H2 weighs two grams, that much oxygen, O2, weighs 32g.
Chemists found this useful, and defined a new concept, the “gram molecular weight.” Which got abbreviated “mole” and got the symbol mol. It’s now an official “base unit” of the modern International (Metric) System, alongside the second, the meter, the kilogram, and the ampere. (There are only two others, and you are about to meet one of those as well.) It’s basically the number of molecules it takes so that the numerical weight of the sample, in grams, is the same as its atomic weight. This is the same number for all pure substances, compounds or elements. We just didn’t know, then, what that number was, but that didn’t mean chemists couldn’t weigh out thirteen moles of copper sulfate when they wanted to.
Even though we didn’t know what the number was, or (equivalently) had no idea how much atoms and molecules actually weighed in grams or kilograms, Avogadro gets the credit for inventing the concept, and that number (now very well known today) is called Avogadro’s number in his honor and is symbolized by NA.
A good set of values for atomic weight became absolutely vital for chemistry. The unsung heroes of chemistry during the 1800s were those who put in years of exacting effort refining atomic weights. Their work wasn’t glamorous, and never would have won them Nobel prizes (if those had existed back then), but chemists knew these guys were doing something Very Important. The biggest “name” here was Jons Jakob Berzelius (who also discovered selenium and cerium oxide), who produced exceedingly good figures by 1826. And in fact people continue to refine the atomic weights, taking into account all sorts of factors we had no notion of until the 20th century.
It became apparent very quickly that atomic weights weren’t quite neat integers. It’s easy enough to quote that hydrogen’s atomic weight is one and oxygen’s is 16, but in fact both numbers are very, very slightly off from those integers, and this was not an artifact of inaccurate measurement. Rather, it’s the way things really are. This must have been maddening for chemists (Why be just a little way off from clean integer ratios? Why not a lot more off from them? It’s like mother nature was shooting at a target and just barely missed the bullseye. Why?)
A pause for an example of using moles.
Chemists making a compound could decide how many moles of it they wanted, for example, say, ten moles. Let’s say our goal is to start with hydrogen and oxygen and to produce ten moles of water. You start out with this idea of the equation for the reaction. It’s really a sort of shorthand recipe.
H2 + O2 -> H2O
Ten moles of H2O is going to contain ten moles of oxygen atoms, and twenty moles of hydrogen atoms, because there are two hydrogen atoms in every one water molecule.
But before you rush off and put 30 moles of gas into a container, there’s one thing to remember. The oxygen going into the reaction is not oxygen atoms, it’s oxygen molecules. And each of those contains two oxygen atoms. So you need five moles, not ten, of O2. And by the same token you need ten moles, not twenty, of H2.
So really, to include the quantities, we should write the equation like this:
10H2 + 5O2 -> 10H2O
But let’s sanity check it. Let’s see if mass is conserved.
Hydrogen’s atomic weight is one. Molecular hydrogen therefore has a molecular weight of 2. So ten moles of this is 20 grams of hydrogen.
Oxygen’s atomic weight is sixteen. Molecular oxygen therefore has a molecular weight of 32. So five moles of this is 160 grams of oxygen.
The total weight of all the ingrediens is 180 grams.
Over on the right hand side, the result is ten moles of water. Water, of course, has a molecular weight of eighteen (one + one + sixteen), and ten moles of it is therefore 180 grams.
The equation seems to balance.
Of course that equation only looks like it does because our goal was ten moles of water. To be generally useful it has to be reduced by dividing through by the lowest common factor. In this case that’s 5, so:
2H2 + O2 -> 2H2O
(One of the things taught in chemistry class is how to balance these equations, like we just did here. In some cases it can get very complicated.)
Any future chemist can scale this up or down, just like working with a recipe that doesn’t make enough (or makes too much) food for your needs.
Let me again emphasize that at this point we didn’t know the mass of any atoms and molecules, and therefore we didn’t know how many were in a mole. But it didn’t matter, we knew the ratios of those masses and could just use moles to keep those ratios consistent.
One last note about atomic weight before we move on.
Because oxygen reacts with a lot of things, and because (unless you are dealing with a gas) you pretty much have to be able to react with something to measure its atomic weight it was convenient to set oxygen’s atomic weight to exactly sixteen, and measure everything in terms of that. So hydrogen’s atomic weight was 1.008 (that’s the best number as of 1949). Much later on we ended up modifying this convention just a tiny bit.
More on Gases…and Heat
As mentioned, a mole of any gas will occupy 22.4 liters at standard temperature and pressure. What happens if you alter one of these parameters?
If you halve the volume, yet keep the temperature constant, you will double the pressure exerted by the gas.
On the other hand, if you double the temperature, either the volume will double and the pressure stays the same or vice versa.
…wait. FULL STOP.
What does it mean to double the temperature? If it’s 20° Celsius, is 40° Celsius twice as hot? Really? Well, 20° C is 68 F, and 40° C is 104 F. But 104 isn’t two times 68.
So it’s only twice as hot if you’re using a Celsius thermometer.
Well, that sure seems stupid, doesn’t it?
We don’t have this problem when doubling mass or halving a length or quadrupling an electric current or waiting for the end of the Biden administration, even if it seems six times longer than it is.
That’s because we can tell what zero mass (or length, or current) is. It’s pretty obvious; if you have none of something, its mass is 0 kg. So doubling the 5 in “5 kg” gives you “10 kg” and by golly, that really is twice as much.
The problem with temperature is that 0° F or 0° C isn’t really “no temperature” or “no heat” in any meaningful sense. What we need to do is to first realize that there’s actually a true zero point to temperature, then figure what it is. Then, it becomes possible to measure with respect to it.
We’re looking to determine absolute zero.
And it turns out we’re already on the right path. We don’t know what half or double the temperature is, but we can figure it out by cooling, or heating the gas until its pressure halves or doubles. And once we know that (just making up numbers) that 559° F is double the temperature of 50° F, we can backtrack and figure out what the real zero point is.
Chemists/physicists did something very much like this. They had to be careful not to let the gas liquefy (all bets are off if that happens), but it turns out that when they plotted the lines, an “ideal” gas would hit zero volume and pressure at -273.15° C, or about -459° F. This is absolute zero.
(I lied. I didn’t just make those numbers up. 50° F is 509° Fahrenheit degrees above absolute zero, so 509 + 50° F = 559° F is twice as hot.)
And chemists and physicists both use a temperature scale that starts at this point, with degree sizes the same as for Celsius (9/5 of a degree Fahrenheit). This is called the kelvin, after Lord Kelvin, an important figure in the history of thermodynamics. In fact it’s not even called “degrees kelvin,” it’s just “kelvins.” This is the sixth of the basic metric units.
300 K works out to 80.33° F, just to help you get a feel for it. And scientists consistently work in kelvins, everything from chemists having to figure out when a material will melt or boil, or how hot something must get before it will react, to astronomers telling you the temperature of Pluto, or Sirius.
As the 1800s wore on, it turned out that, deep down, the temperature of an object was directly related to the average kinetic energy of the molecules inside it. The total energy of the heat in the object is of course the sum of all the molecules’ kinetic energy, or in essence the total kinetic energy inside the object. But now we knew what heat was…it’s actually a manifestation of kinetic energy. And this is why when friction occurs objects heat up; the energy of motion is being transferred to the individual molecules. The object as a whole slows down, but the molecules start moving around with respect to each other (picking up the momentum the object loses, remember momentum is conserved) and the object heats up.
Humphry Davy
We now turn to the other thing that was going on starting in the 1800s (this time I don’t mean the century but rather the “zero years” of that first decade). I mentioned this in passing in part IV.
Sir Humphry Davy (1778-1829) exploited the voltaic pile (battery) to bust apart molecules that had been impervious to other methods (a typical method was to try to bring oxygen in to grab one constituent of a molecule, since oxygen is very good at “cutting in”).
The basic procedure was to prepare a solution of whatever it was you wanted to break apart, stick two electrodes into the solution, connect them to a battery, and wait for the electricity to do the work. One part of the molecule would collect around the positive electrode and the other part around the negative electrode.
Apparently, moving an electric charge around could induce at least some molecules to break apart.
Convinced that potash contained an undiscovered element (in spite of Lavoisier not thinking so), Davy made up a solution of it in water, hooked up the electrodes, and got hydrogen and oxygen. Whoops. He was busting up the water. But he needed a liquid for this to work. So he tried molten potash, and that worked like gangbusters. There were flames at the negative electrode. Taking a closer look, there were globules of silvery metal forming there, which would immediately burst into flame, just from contact with the air.
Davy was able to capture some of these globules before they self-torched and tried putting them in water. They’d race around the surface of the water and burst into lavender light. It turned out that the water was being broken apart into hydrogen and hydroxide (OH) and the hydroxide was reacting with the metal, to form KOH (potash lye). The hydrogen, on the other hand, was hot enough to spontaneously combust to form water vapor. Whatever this new stuff was, water would burn it!
According to witnesses, Davy danced around the laboratory with joy. He had just discovered potassium.
He tried soda (no, not coca cola). It took more voltage (electrical potential, the push) but he isolated sodium in short order. Sodium, of course is now famous for pyrotechnics when put into water. (It’s very, very dangerous, by the way, to simply throw a piece of sodium into a lake–a jet of hot, fresh soda lye (NaOH) might just shoot out the way the sodium came, land on you and blind you. However, I can promise Barry Obola that he is so anointed that he will come to no harm whatsoever if he does this. Trust me, Barry.)
Davy also nabbed magnesium, calcium, strontium and barium, elements that Lavoisier had identified as being there without them having been isolated. With the exception of magnesium, these would all spontaneously react with air and moisture energetically. Magnesium, the one metal that didn’t, was barely a successful find; it turned out a more successful method of isolating it was to react one of its compounds with pure sodium, so as it happens Davy was a key part of that effort anyway.
Davy had even more trouble with lithium; only small, wretchedly contaminated samples resulted from his efforts, and indeed it wasn’t until 1855 that good samples of lithium were isolated.
Michael Faraday (again)
All this was in 1807-1808, but Davy wasn’t done contributing to this story.
In 1813 he hired Michael Faraday. Yes, that Michael Faraday. The Michael Faraday, who alongside Newton and Maxwell, had his picture hung in Albert Einstein’s office. The Michael Faraday from last week that you were supposed to thank the next time you flipped a light switch (did you?).
Given that Faraday never had formal education, and learned all his science on the job, Davy did the world a tremendous favor giving him a chance. (So thank him, too, the next time you flip a light switch.)
As if unifying electricity and magnetism and laying the groundwork for modern civilization weren’t enough, Faraday also investigated electrolysis, following in Davy’s footsteps. In fact, he invented the words “anode,” “cathode,” “ion” and “electrode.”
Faraday is responsible for the discovery that in order to break a single bond, like say that between sodium and chlorine in salt, with electrolysis, a certain amount of electrical charge has to be supplied. And this number was the same per bond, per mol. This is, in fact, Faraday’s Constant.
To break one mole of single bond, it required 96,485.3 colombs. (Remember, once again, how humongous an electric charge one coulomb is.)
If it was a double bond, it would take twice as much charge.
This alone should be enough to convince anyone that there is a lot of electrical charge in simple, ordinary materials. We never noticed because it’s almost always perfectly balanced. When it falls out of balance, your sheets stick to each other coming out of the drier, your cat gets covered in packing peanuts, balloons pull your hair into a mess, and so on. On the plus side, if you can get the electrical fluid to move (without causing a huge imbalance) you can get it to work; a lot of work.
You could even think of this number as a mole of electric charge, since it operated to break one mol of single bonds (or half a mol of double bonds).
Chemistry, it was becoming quite apparent, is actually an electrical thing. Remember when I said, last time, that electricity is responsible for every physical phenomenon you see around you, except for gravity? That included things like why it’s hard to break rocks (electrical forces keep the rock bonded to itself), why water takes as much heat as it does to boil, anything having to do with light, and on and on. It includes things set on fire. It includes the question of why you and I aren’t just loose piles of disorganized atoms.
Dmitri Ivanovich Mendeleyev
(A quick linguistic note. Mendeleyev’s name is properly spelt: Дмитрий Иванович Менделеев, but I suspect most of my readers can’t read Cyrillic, so it’s necessary to transliterate his name into the Latin alphabet. Usually when this is done, the “y” is not included, but I think it’s better to use the y, because it is most definitely pronounced when English speakers pronounce his name (and for that matter is implicit in the second of the pair of еs in the original Russian). Those in the know know it’s “men-del-A-yev” rather than “men-del EVE” (it’s probably a way of hazing noob chemistry students who don’t know the trick and blunder) but the most-common transliteration doesn’t reflect this. Since the transliteration is supposed to be helpful, I decided to use the more-helpful, less-common alternative here.)
I started this article by pointing out that chemistry was a collection of unsorted trivia until Lavoisier, who finally got us on the right track to figuring out what substances were compounds, and which ones were elements, the basic building blocks of everything you can drop on your foot.
But Lavoisier knew of thirty one elements. By 1869 there were sixty three of them (including one mistake, didymium, that was really two elements that today we call praseodymium and neodymium).
This is an awful lot of different basic building blocks, isn’t it?
There seemed no rhyme or reason to it. Most of their masses were almost, but maddeningly not quite, integers, but even ignoring the tiny fractions, the numbers were chaotic. In order, hydrogen 1, lithium 7, beryllium 9.4, boron 11, carbon 12, nitrogen 14, oxygen 16, fluorine 19, sodium 23, magnesium 24 for the first ten.
What went into the holes? Was there something with an atomic weight of almost-but-not-quite 2, 3, 4, 5 or 6? What was up with beryllium?
Some chemists had begun to notice that some elements seemed chemically similar, for example, fluorine, chlorine and bromine, or copper, silver and gold, or chromium, molybdenum and tungsten. There seemed to be a lot of “triads” of elements like this.
But it was Dmitri Mendeleyev (1834-1907) who was the first to perceive the entire pattern…and to put a lot of confidence into it.
He sorted the elements according how they combined with oxygen. The first group (hydrogen, lithium, sodium, combined 2-1, two atoms of the element to one of oxygen. Each of these took up one of oxygen’s two bonds. You can write a generic formula, R2O for this. And to make the pattern clear, figure that an average atom of the first group combined with one half of an oxygen atom.
The second group was one-for-one. Beryllium, magnesium, calcium all took up both of oxygen’s bonds, generic formula RO.
Then there was a two-to-three group, boron, aluminum, etc, where two atoms of the element, with three bonds apiece, would combine with three atoms of oxygen, for a generic formula R2O3, or each atom combining with one and a half oxygen atoms.
This could be carried through until you got to elements that would combine with four full oxygen atoms (RO4), giving a total of eight possibilities, with elements sorted into eight groups.
Mendeleyev could sort these groups each by increasing atomic weight, then set these groups next to each other as columns in a grid. When he did that, he could read across, from group 1 to group 8, increasing atomic weights in the top row. Then the next row started in group 1 with a higher atomic weight and repeated the process. It was a periodic trend, every eighth element landed in the same group.
There were a few irregularities. For instance group eight, the one-to-four group, would either be empty on a given row, or hold three neighboring elements (iron-cobalt-nickel, ruthenium-rhodium-palladium, osmium-iridium-platinum), which was a bit of an irregularity, but it was a regular irregularity as every other row had one of these triples in column 8; the empty cells and the cells with three elements alternated.
That was far less interesting than some of the other irregularities in the sequence. For instance calcium belonged with beryllium and magnesium above it (and strontium and barium below it) in the one-to-one column, column 2. But the next element after that was titanium, which was a two-to-one which did not belong in the next three-to-two column which had boron and aluminum. Rather, it belonged better in column 4. So maybe this was all a waste of time?
Or maaaaybe the cell skipped over was a hitherto unknown element! So leave that spot open, and put titanium under carbon and silicon, the one to two column, where it belongs. (Titanium dioxide is a thing.)
There were two more holes between zinc and arsenic. And others, but Mendeleyev chose to focus on these three.
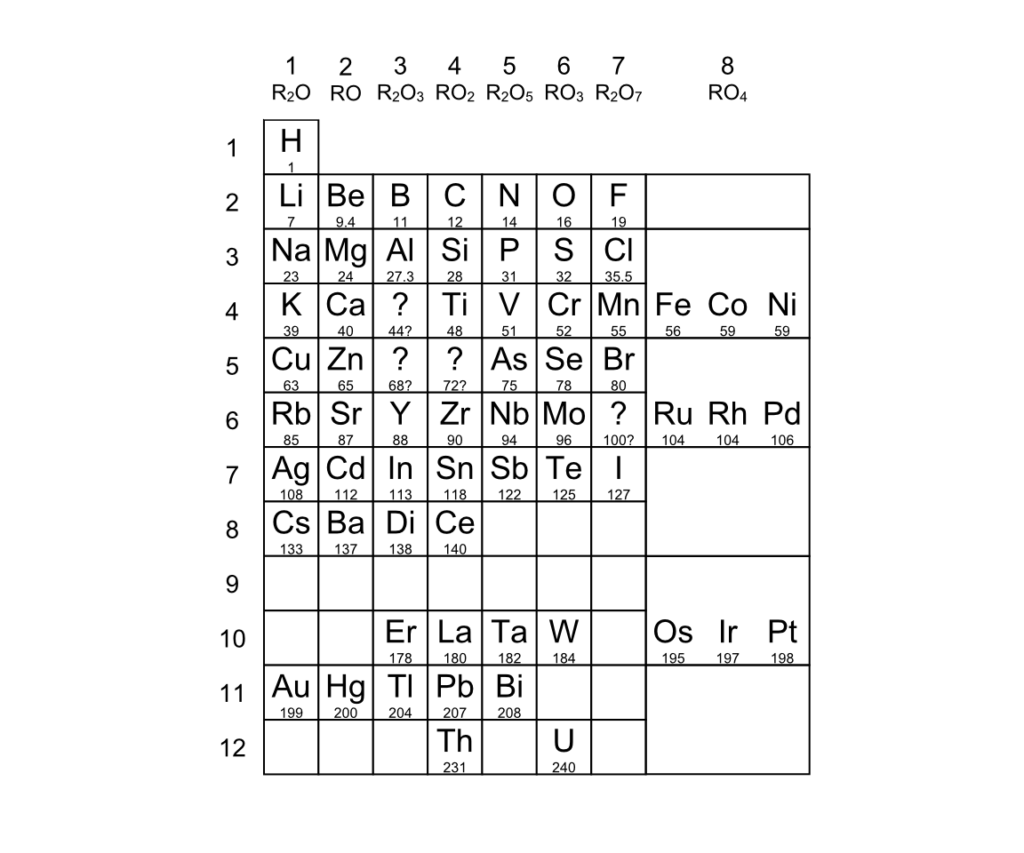
He wrongly placed Di, Ce, Er, and La (rows 8 and 10). Di (didymium) turned out to be two different elements,
but really La (lanthanum) should go in that square, not either of the two hiding in “didymium.”
Mendeleyev predicted three new elements to fill these holes. The first one he predicted an atomic weight of 44, an oxide R2O3 weighing about 3.5 grams per cubic centimeter. He made other predictions for the other two elements.
All three of these elements were found in the next 20 years, they are scandium, gallium, and germanium respectively. And they matched up with Mendeleev’s predictions pretty damn well. Not exactly, but far too close to be random chance.
Mendeleyev was definitely onto something. Previously, elements had popped up at random, with no rhyme or reason, totally unpredictably. A bright chemist might have a hunch that some mineral (say) had something new in it, and might even be able to prove it without isolating the element, but one could never tell when such a thing would turn up, or what the new element would be like, until isolated.
But now Mendeleyev could tell you, before anyone else had so much of an inkling as to the existence of an element, what it would be like!
Because of this, it didn’t take long for chemists to accept this pattern. It’s now called the periodic table of the elements. It has gone through several changes (the most important going from 8 columns to 18, or actually, 32) but it traces right back to Mendeleyev. It became so deeply ingrained, that chemists were even willing to disregard atomic weights if they were out of the periodic table sequence. In particular, 1889 a chemist named Brauner measured the atomic weight of tellurium very carefully and got a higher value than before, 127.6. This was a group 6 element, in the column headed by oxygen. Its next door neighbor in group 7 was iodine, and iodine had an atomic weight of 127. So now all of the sudden, tellurium had a higher weight than the next element in the sequence.
Does this mean that iodine and tellurium should swap places? Nope. Leave them where they are. There must be some reason for the oddity, but matching group membership was more important than arranging things in order by atomic weight. (Mendeleyev’s attitude was a bit different. He apparnetly figured the new number for tellurium must be mistaken; he wasn’t willing to part with the assumption that the atomic weights had to increase as you read across the rows, but he clearly did think the periodic sequence was more important; given a “contradiction” he went with the periodic table, not the atomic weight data.)
But even as the periodic table was being accepted as an organizing principle, it looked like it was starting to unravel. In the early 1800s chemists started discovering “rare earth elements” with atomic weights between 138 and 175. (No other elements were in this big gap.) They found more and more of these elements…and they were similar to each other, enough so that they were hard to separate, and the similarities were in fact why newer elements were able to hide within older ones. It’s like they were all trying to cram into the square below scandium and yttrium! (Mendeleyev knew of four in 1871, there would ultimately turn out to be fifteen of them.)
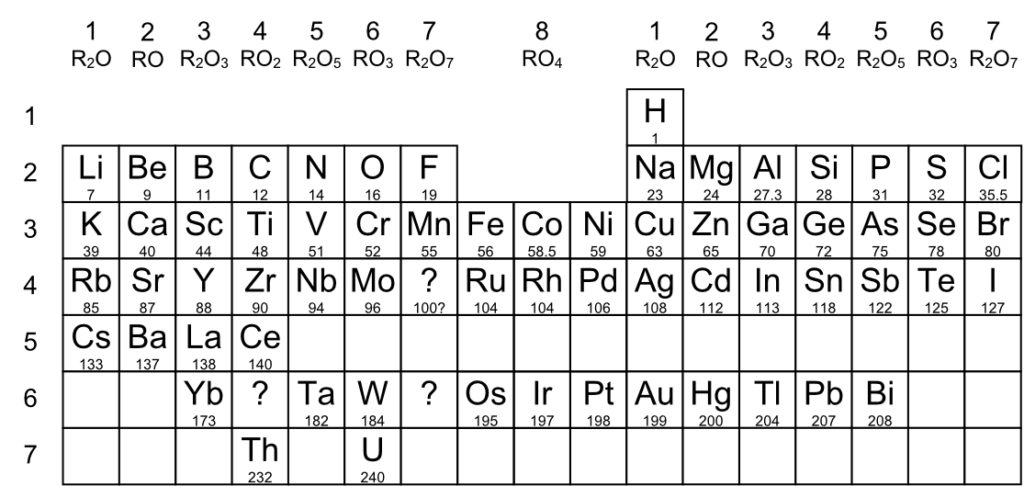
This is probably a bit more recognizable to modern eyes; rows 3 and 4 are almost dead-on as today’s rows 4 and 5.
The following rare earths are not included: Er (erbium), Tb (terbium), Ho (holmium), Tm (thulium,
Sm (samarium), Gd (gadolinium, Pr (praseodymium), Nd (neodymium), and Dy (dysprosium).
Nd and Pr are the two elements that had previously been combined as Di.
Ce and Yb are not on the right places (they are rare earth metals).
As more and more of these elements were discovered, Mendeleyev simply didn’t know what to do with them and just gave up trying to fit them in–leaving it for a future genius to solve. Other chemists tried to organize them and failed to do anything convincing with them. Since they didn’t follow the rules, there wasn’t even any way to know for certain how many of them there were!
So it was frustrating. There was only partial order to the elements, but then, where there was order, it was very, very useful. Call it a win, overall, even if it wasn’t a rout.
Sir William Ramsay
In fact, there wasn’t even any assurance that there wasn’t a totally unseen column in the table.
Wheatie asked me the question, once, as to whether there could be undiscovered elements between the ones we know about. Without pulling in a spoiler, the answer is basically “not no, but hell no!”
That’s the answer today, because of discoveries made in the 1910s. Back then, that was not the answer by a long shot; there were known holes in the table such as Mendeleyev’s three predictions. And who the heck knew how many of those damn rare earths there were, not to mention more holes like the one at atomic weight about-a-hundred.
Well, back up to 1785. Cavendish…remember him? G? Flammable air (i.e., phlogiston hydrogen)?
In a totally different experiment, Cavendish had reacted phlogisticated air (nitrogen) and dephlogisticated air (oxygen) with a spark, repeatedly, making niter. But some of the nitrogen just wouldn’t react. Since his source for these gases was the atmosphere, he was able to determine that this residue accounted for 1/120th of the atmosphere. (He was a very careful, meticulous and precise measurer, which is how he was able to determine G, a difficult thing to measure even today.)
And there that matter stood, basically forgotten, for almost a hundred years. Until 1882 when Lord Raleigh at Cambridge University’s Cavendish Laboratory (the irony!) was working with hydrogen, oxygen and nitrogen, trying to determine their densities and hence (thanks to Avogadro’s law) their atomic weights. He got good solid values for hydrogen and oxygen, but for nitrogen, he couldn’t get consistent results. If the nitrogen came from ammonia, his result was 1/2 of a percent lower than if the nitrogen came from the atmosphere. Raleigh was probably banging his head on the wall in frustration. He wrote to Nature, the preeminent scientific journal, asking if anyone else had any idea what was going on, just like today we might post on a chemistry forum online. He got a bunch of suggestions, including that the leftover gas might be N3, a hypothetical, less reactive form of nitrogen, just as oxygen could form O3 (ozone) instead of its usual O2.
Sir William Ramsay took another approach. He took some air, passed it over hot copper to remove the oxygen, hot magnesium to get rid of the nitrogen, soda lime to get rid of the carbon dioxide, and phosphorus pentoxide to get rid of the water vapor.
What he had left was about 1/80th of what he started with. At first he and others thought that this was indeed N3. But Sir William Crooks was able to prove that whatever this was, it wasn’t any kind of nitrogen.
In 1894, Ramsay realized the truth. This was a new element, one that didn’t react to oxygen at all. For that matter, it didn’t react with anything else either, including itself. This was argon, and it’s in every breath you take. An utterly non-reactive gas.
In addition to group 1, where every atom reacted with half an oxygen atom, through group 8, where every atom reacted with four oxygen atoms, in steps of half an oxygen atom, there was something one step to the left. Atoms that would react with no oxygen atoms.
This explained what Cavendish had seen.
There was a whole new column in the periodic table, call it Group 0.
Ramsay continued working into 1895 looking for other members of this column, unaware that he’d been partially scooped.
But 1895 is our line. We’re not quite yet ready to step across it.
Conclusion
There’s no new conservation law this time, rather a reinforcement of the conservation of mass and the conservation of energy, but we have plenty of mysteries.
Why are there so many different kinds of atoms? It’s nice that they form a pattern, but it’s not a perfect pattern, and those damnable rare earths really bork it in one place. Why is there a pattern, and why is it not perfect?
What is the relationship between atoms and electricity? We still don’t know what the electric fluid is. We have one tantalizing clue, that a bazillion coulombs (okay, 96,485.3 colombs, but that’s a lot) of charge seems able to bust up one mole of a single bonded molecule.
Remember, as far as we knew, an atom was an indivisible thing. Yet they seemed to be swapping electrical charges (or something) when forming compounds, with electrolysis somehow undoing that to break compounds apart.
All of which just pointed to a need to keep investigating atoms.
Obligatory PSAs and Reminders
China is Lower than Whale Shit
Remember Hong Kong!!!
中国是个混蛋 !!!
Zhōngguò shì gè hùndàn !!!
China is asshoe !!!
China is in the White House
Since Wednesday, January 20 at Noon EST, the bought-and-paid for His Fraudulency Joseph Biden has been in the White House. It’s as good as having China in the Oval Office.
Joe Biden is Asshoe
China is in the White House, because Joe Biden is in the White House, and Joe Biden is identically equal to China. China is Asshoe. Therefore, Joe Biden is Asshoe.
But of course the much more important thing to realize:
Joe Biden Didn’t Win
乔*拜登没赢 !!!
Qiáo Bài dēng méi yíng !!!
Joe Biden didn’t win !!!
No way I’m first!
I’m wrong about that, apparently.
And I did read the post, but I cheated, by proofing it. 😉
Happy Caturday!

LOL!
Dogs need their own day of the week. Honestly.
Every day is Dog Day in my house. My dog makes sure I don’t forget.
5:30 am Ying got the house up. An hour later, she’s asleep.
Same. They wake up all refreshed and then it’s “let’s go do something!”
Ying just wants her people around her. It’s a Havanese trait. She’s also mad at me. I took her to the vet Thursday, and her ears got massively cleaned out. There was an infection I just couldn’t beat, and I think it’s yeast, so it’s been cultured. We’re probably looking at anti-biotics and anti-fungals.
Yuck.
And a knee problem, and arthritis, and she needs to lose weight. And….
Have a very needy cockapoo with yeast in her ear and a trick knee. Entederm once daily in the ear. Seems to be working.
LOL @ “needy cockapoo”!
Sort of like the Golden in this one (now that I think of it, the Lab) 😆
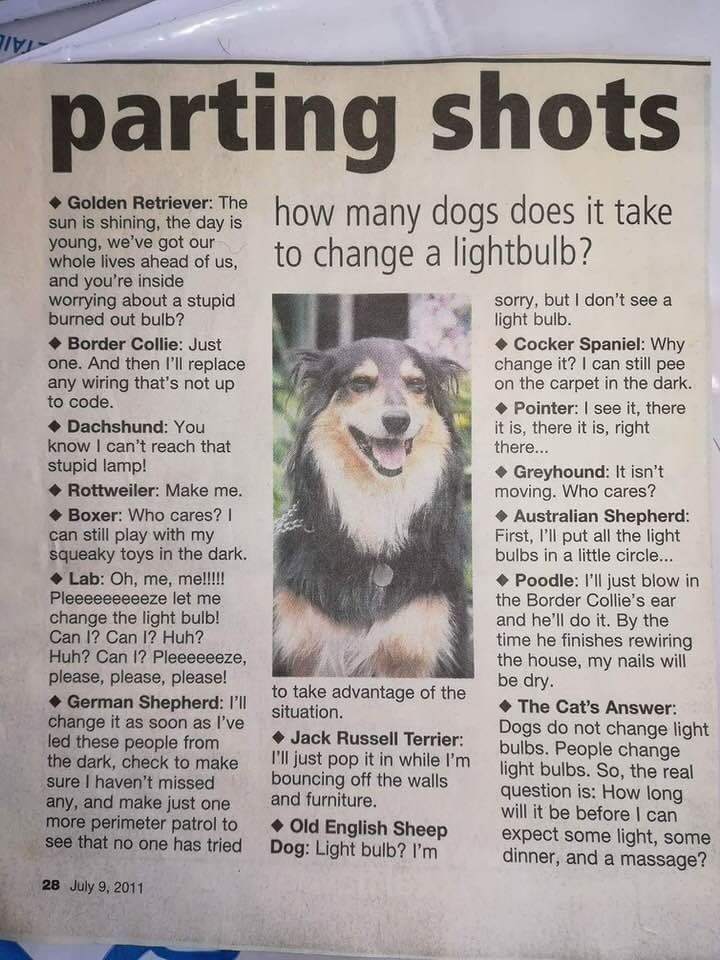
LOL That’s great! Going to pass it along to some fellow dog lovers.
I have a sixteen-year-old pup who gets us up at the crack of dawn every day. He won’t let us sleep in.
My foxhound waits as long as shes gone out 2 oee once or twice at night.
We’ve had her 15 months now and still has not barked or howled except during a dream.
Ooh. Foxhound! I’m going to take a look at them.
She is a good dog. Getting fat and lazy being a pet, but only 60 lbs. Not a digger, getting on furniture, or chewer.
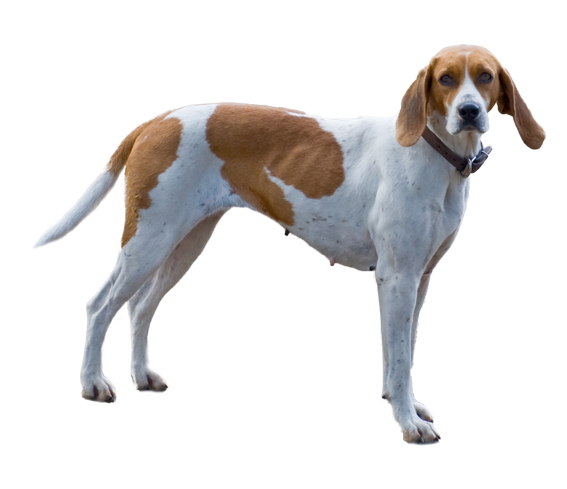

Her color is tan and white.
Muttday?
Cursday?
Fidoday?
Or maybe Caturday needs Dog Day Afternoon! 😃
It will be a dog day today. WHEW! It’s going to be warm.
I have often noticed the first comment on a post is at least 20 minutes after midnight, so this is early.
I have not checked the comments yet to see if anybody other than Cthulhu appreciated the rather amazing compression of “advancement of the elements from 4.5 to N” into a short and very understandable “de-Vulcan-ization of phlogiston”, but good show!
I will get it into the sidebar soon enough!
Thanks!
Done!
Kyle Becker (@kylenabecker) Tweeted:
BREAKING. 🚨
PENNSYLVANIA has unveiled an Election Integrity bill that includes Strict Voter ID. This is *major.*🔻 https://t.co/jG6MKmWlr8
The last time anyone here has to show ID is when they first register to vote. For me, that was 20 years ago when I moved back here. /smh
Wow – that’s nasty. We have to show ID every time we vote!
Maybe that’s why we only have communists working as bartenders, and not running the state!
Now you can understand why Oregon hasn’t had a republican governor since mail-in voting started.
This has to end. We cannot let this continue.
It’s such an outrage. Just such an outrage.
We do have to show ID, if and when we vote in person. Unfortunately we are encouraged to mail in our ballots.
But then it turns out that an acceptable form of ID is a utility bill.
We have to show an ID to buy cigarettes & booze. We had to show it for ONE election then the Demon-Rats challenged it. It went to the Supreme Court but was never defended by our state AG… GUESS WHO HE WAS??
Yeah, that is right COOPER who then won the governorship in 2016 by having more votes than voters in 20 of our 100 counties. 🙄 😫
Walk into an inner city neighborhood and claim that a bunch of them have no ids and therefore wouldn’t be able to vote…the people there will laugh in your face. They need an ID to do anything (cash a check, buy legal drugs (alcohol and nicotine), you name it…).
They’re more likely to have to show ID than middle class people in fact.
Make it as hard to vote as it is to buy a gun.
I like that.
Or at least as hard as it is to buy BOOZE!
Don’t get excited. The Democrat Governor has already said he’ll veto it. Not enough votes in the Legislature to override.
https://www.msn.com/en-us/news/politics/pa-gov-tom-wolf-says-he-will-veto-any-bill-that-suppresses-voter-access/ar-AAKSLQI
No problem. Make them show their cards before the final fight.
BREAKING: AG Merrick Garland Announces that His DOJ Will Scrutinize Any Post-Election Audits for Evidence of Voting Law Violations!!
June 11, 2021, 3:03pmby Joe Hoft 4134 Comments
https://www.thegatewaypundit.com/2021/06/breaking-ag-merrick-garland-announces-doj-will-scrutinize-post-election-audits-evidence-voting-law-violations/
Oh, I cannot say FUCK YOU, DODGE in strong enough terms over this.
YOU LYING SACKS OF SHIT WILL RETURN THE DIAMONDS.
OR AMERICA WILL SEE YOU HANG.
“YOU LYING SACKS OF SHIT WILL RETURN THE DIAMONDS.
OR AMERICA WILL SEE YOU HANG.”
____________
Umm, I’m going with AND LOGIC for that one…. 👍😁
Trump did an amazing thing by letting the crooks leave the property with the stolen diamonds without being shot at.
NOW we see who is real and who is not.
Good GOD almighty – to see DOJ threatening AGAINST truth and justice – it is an absolute abomination.
To see the DOJ and FBI engage in criminal behavior is now an outrage that demands justice at levels not seen in thousands of years.
Merrick Garland, how can you be part of this? How? Have you turned against GOD HIMSELF?
“Merrick Garland, how can you be part of this? How? Have you turned against GOD HIMSELF?”
_______________
If he hadn’t, would they have ever hired him?
I guess that’s a “yes no matter what he says”.
He’s a DEMONRAT, and the DEMONRATS threw GOD HIMSELF out of the DEMONRAT “party” and the DEMONRAT “platform”.
The party of abortion, perversion, crime, and “situational ethics” has no place for nor any interest in any god other than themselves and their master, Satan…
Bid – Ho, Bid – Ho, it’s off to Hell they go…
Was Merrick Garland ever with God in the first place?
It’s a great question.
Wiki avoids any mention of religion, but the question was asked when he was nominated by Obama.
https://njjewishnews.timesofisrael.com/inside-the-jewish-life-of-supreme-court-nominee-merrick-garland/
He’s on an arc of decreasing rigor but plateaued (raised Conservative, married Reform, member of a Reform congregation). Family history looks potentially “diaper”.
Interesting story about Reno and Ted Kaczynski and a seder – there is some interest there – a bit too complicated to get into now, but something interesting.
THIS statement he made at the time of his nomination to SCOTUS borders on hilarious in the current context.
“I think the rule of law is what distinguishes our country from most other countries,” Garland says in the video. “It’s people’s willingness to trust that they don’t have to take justice into their own hands, that law will treat people fairly and impartially, and without regard to politics or religion or race or anything else.”
REALLY.
COUGH.
I think he’s really, REALLY PWNED, by someone (Klintoons, Kabal (lah),
Øbløwhøle, etc.). That the DEMONRATS would take out Scalia so they could deposit him onto SCOTUS speaks volumes. Dark and witchy tomes…
Replacing Scalia with deep diaper makes loads of Obama sense. Garland is core Chicago, too. Makes loads of sense.
I truly believe that they have.
Or as it says ” I will have mercy on whom I have mercy, and I will have compassion on whom I have compassion.”
There put in place to carry out evil,
“Therefore God has mercy on whom he wants to have mercy , and he hardens whom he wants to harden.”
If they made up votes for the 2020 election, they will absolutely make up false ‘evidence’ of post-election audit ‘tampering’ to preserve the fake 2020 election result.
It’s the equivalent of the police showing up during an armed bank robbery, and now the robbers are taking hostages.
They will threaten to kill the hostages.
And then they will start killing them, one by one.
The one thing they will never do is surrender, because they know they’re dead either way.
It’s how every such situation terminates.
It reaches a point where the Police have to rush the bank in order to save as many hostages as they can, because if they don’t, all the hostages will be killed.
That is where this was always going, since 2016.
There was never any end-game that did not involve physical force to stop the global crime syndicate, because the global crime syndicate knows that if they give up, they’re dead.
As long as they don’t give up, they’re still alive.
Alive beats dead, ergo they will never give up, ergo the only way to stop them is to stop them dead.
From the very beginning, that was how the story ends.
We’re just waiting for the discovery of something they’ve done that is so heinous, the people can’t even hear the narrative anymore, because they’re rushing en masse at the criminals, everywhere, at once.
It’s going to be interesting.
I tend to agree.
This is one massive uncovering of corruption. I still reel from it at times.
It is absolutely surreal.
They won’t go peacefully. They’ve gotten so used to cheating and lying that they feel it is their right to usurp power from the people.
This is their moral trick. As long as THEY are able to do it non-violently, they say “you can’t use violence against us”.
But when the chips are down, they let SOME of their own use violence, and they IGNORE IT.
It’s evil. It’s utterly evil.
And it is so arrogant! It makes me grit my teeth in anger.
Same here. It’s not America. It’s not even MEXICO.
“This is their moral trick. As long as THEY are able to do it non-violently, they say “you can’t use violence against us”.”
_____________
No violence, just arrest.
If they resist arrest, then they commit the violence, and we’re only responding to it with necessary force.
“To everything, there is a season…”
“Violence is never the answer.”
vs
“Not only is violence sometimes the answer;
Sometimes violence is the only answer.”
Indeed.
I’m tired of theses over-simplified catch-phrase mantras, used to dumb-down gullible people.
They truly are *spells* … and they still WORK on far too many people today (as opposed to decades, or even centuries, ago).
“Romantic semantics”
😉
“Non-violent” is itself the wrong concept and we shouldn’t play into it.
Another aspect of this perhaps overlooked is that they will actually use force in, for example, invading someone’s office but then staging a “sit in.” (Or the modern manifestation; “occupying.”) The “sit in” is, by itself and out of context, non-violent; they’re not breaking anyone, they’re not engaging in battery. But the fact is they forced their way into a place they have no right to be and are refusing to vacate, and that is a use of force.
Passive-aggressive.
Or, my favorite, obsessive-repulsive…
There’s gonna be a fight. I don’t see any way out of it now.
Yep. However, once the dust settles we may have our republic back.
I hope the good guys are ready.
Yes. We shall have it back.
This kind of criminality by the DOJ is intolerable.
The DOJ behaves like the Stasi.
They try to instill fear and control through fear and threads and then make examples out of some high profile people. When they make the example they count that no one ever questioned their authority.
This needs to be slapped douwn right away and they cannot have success with their tactics.
Mistake that non of these crimminals got jailed under Trump.
Yup. Indeed, it seems like THEIR “Plan B” was to allow Trump into the White House and then destroy him there – and when that failed – destroy him AND his movement in 2020.
These people are INSIDIOUS.
I think you are right !
They are pushing us to see how far they can go.
Agreed, that’s been their end game all along. Get the people to resist then they can come and clean up the rest.
“In the name of Law and order.” 🤣😂😅😆
Just sad. Bolsheviks. They never change.
No reason to fix what works.
“they will never give up, ergo the only way to stop them is to stop them dead.”
Yes.
Exactly. Things are so unevenly set up, with crooked lawfare, crooked judiciary, corrupt upper level military, etc….I’m sure that none of them are even afraid of exposure. Maybe a little but really who’s going to arrest them, jail them, try them ?
Will it only be the lowly techs ? Until the rot is cut off and burned none of this will improve.
It’s on Weiner’s laptop and involves H.
“There was never any end-game that did not involve physical force to stop the global crime syndicate, because the global crime syndicate knows that if they give up, they’re dead.”
ASSUMPTION: The above statement is true.
QUESTION: Why did Trump let it go this far?
QUESTION: Why did no one with legitimate authority (government officials) do anything in the face of an obvious treasonous act knowing that taking no action would lead to an inevitable deadly solution?
QUESTION: Why did institutions designed to address these specific problems stand aside or collaborate with the traitors?
It is now a much bigger problem than it would have been six months ago and will, if the chosen strategy remains to do nothing, continue to grow until civil war is no longer a possible solution but the ONLY solution.
Yes and military men invade our border. On whose side are they going to be?
Every once in a while, the possibility occurs to me that:
A) we have been in WWIII since the beginning of the DJT administration
B) the corrupt government members were given a choice to fight for America by turning their normal subversive role up to ’11’, to expose everything by ‘going over the top’, to the point that it could no longer continue… and they accepted
C) and in doing so, force the issue and overthrow the CCP takeover and control of the United States, which in turn
D) would expose and overthrow the CCP takeover and control of just about every Western country in the world
It’s just a dream I had… 😁
“Justice Department”. AS IF!!!
“Earlier on Friday, the Biden Department of Justice warned state lawmakers that if they proceeded to audit fraudulent elections they will be targeted by the Biden administration.
The US Constitution specifically gives the power to the states to regulate federal elections as they see fit.
You would think the Attorney General of the United States would know this.
Following the threats by the Biden regime, former fighter pilot and Arizona state Senator Wendy Rogers warned Merrick Garland to stay in his lane…..”
https://www.thegatewaypundit.com/2021/06/arizona-lawmaker-responds-ag-garland-will-not-touch-arizona-ballots-machines-unless-want-spend-time-arizona-prison/
The elites have bit off more than they can chew.
The diamonds must be returned, or the dirty cops will need to be arrested.
This is where SHERIFFS COME IN!
Wyoming Sheriffs Told Federal BATF & IRS Agents To Abide By The Constitution Or Face Immediate Arrest. UPDATED!
SO THERE IS A LEGAL PRECEDENCE ALREADY SET!
I agree – sheriffs are the key.
AFAIK, a County Sheriff has the power to arrest even the State Governor, if that Guv is in his / her county.
The same goes for the USAG – like we’ve just seen in the current spat in AZ, except it’s the AZ State AG in this case.
Perhaps we need a mail (et al.) campaign to inform / remind all 3000+ County Sheriffs of their duties – and powers – in this regard.
A little gray area…
The US attorney general is appointed by the chief executive (after being confirmed by the Senate).
State Attorney Generals are elected.
(although they both like to tout the idea they execute the duties of the office “without fear or favor”).
>>”A little gray area…”<<
Yeah!
My analogy of Sheriffs of Counties and AG’s of States (and of the Country) isn’t as exact as I could have made it – but such is the nature of analogies, always part right and part wrong.
The central idea is sovereignty – and it seems to be that County Sheriffs have a good amount of it (over State officials AND Federal officials), just as (almost!) State officials have a good amount of it over Federal officials.
There are exceptions, of course – but they are exceptions (minor) rather than rules (major).
Yes… Perhaps more accurately, “checks and balances” ?
(as I’m sure that there is such thing as “rogue sheriffs”)
😉
Can Sheriffs deputize citizens to help them if the Federal Government comes in trying to bully or overstep their authority?
>>”Can Sheriffs deputize citizens”<<
Yes.
At will (their discretion is absolute, in effect), and without any limit as to the number of individuals so deputized.
I for one would like to see it implemented ALL OVER our beloved American Constitutional Republic.
Let’s “Git ‘er done”! Haha …
Good there will be a serious fight if Garland oversteps his authority. They will put the military against the citizens.
Now it makes sense why they demonize deplorable in the FBI.
They are too late to the game of having troops fire on civilians…at least in a big way.
They do not have the rank and file.
I think PT made them pull the trigger too early. This is, perhaps, why they have gone bonkers trying to implement CRT and associated insanity.
Pray you are right 🙂
😇🙏
Ah, this makes sense. Wow.
Thats why gruesome hasnt left his county except for hidden flights to montana.
Garland has shown himself to be a communist thug.
We might have a serious fight between state and federal government if Garland steps over his line.
I’m thinking he may be deep diaper.
And he’s fixing to have a “racing stripe” (brownfog day) in that diaper if he keeps up with what he thinks he can do…..
Hi Miss Sylvia. Hope the wound scar is starting to fade a bit by now and no complications.
From GAB, from Flynn’s Telegram account, copied from Twitter:
People are FINALLY standing up to these corrupt bastards.
“The Justice Department is one of the most corrupt institutions in the USA.”
Yep, here we are. States are taking back the rights granted to them by the Constitution and usurped slowly for over a century by the federal government.
It almost seems like a plan or something… 😉
Sure hope so.
This is very gratifying to see. The pushback on these criminals.
It’s just so NAKED and RAW now – now that they’re holding power and feeling like nothing can stop them.
People can SEE IT. We HAVE to push back. We MUST.
It’s naked and raw because VSGPOTUSDJT pantsed it.
LOL! Yup.
Everybody circle up around them now and chant with me:
“I see your weiner, I see your weiner!”
Just his laptop will do (Nick Danger’s, that is)…
“I see your TINY weiner”, followed by the name of a specific government criminal, would be even more hilarious 👍 😁
I suppose they’ve gamed out scenarios where states won’t fall in line and citizens push back. I mean the January 6 rally is their jumping off point to shut down citizens expressing their rights.
None of them have an ounce of patriotism left. They’ll do what it takes to stay in power.
Everyone should be prepared to hunker down.
Prepare for all possibilities. Hunkering down is one, but if a grand army of restoration happens, I’m there.
I train fast, and I wouldn’t mind driving a TANK headed for DC.
My truck made its last trip there. I need something with more firepower.
We have a COMMUNIST INSURRECTION to put down.
NICE!!!
“Everyone should be prepared to hunker down.”
___________
Or hunker up!
😁 👍
I’d say she’s got his number.
The states touring the audit in AZ include Washington, Utah, Missouri, Oklahoma, Nevada, Virginia and the ones in contention.
Things are going to get sticky if the fake administration tries to stop all this.
Yes. “The diamonds must be returned.”
When the cops hold the diamonds, and come up with excuses, they go to the top of the real “Ten Most Wanted” list.
Andrew Torba https://gab.com/emoji/271d.svg
@a12
h
·
I want to see clips of based moms making fools out of school boards and based dads making fools out of city council members daily. We need to keep this going. The real movement is in your backyard, not in Washington DC. Federal politics is subverted by corporations and foreign nations, but for the moment we can still regain control of our own local governments and schools. It’s time to do so.
4,341 likes
215 comments
1,557 reposts
Smidge of reality down San diego way about the covid lockdown. Gruesomw and his cronies deserve prison but some reality and life hs pushed him aside “whether he likes it or not.”
KUSI News (@KUSINews) Tweeted:
California politicians continue to play the color-coded game, but most people living in the “real world” are not.
“Let’s just be real, places have been open, and this whole color-coded thing has been ignored for a long time.”
Full Story: https://t.co/v19ahsLmV7 https://t.co/Nw3xFbEH8D
Its not us the conservatives. We dont want this. Its a bottomless pit, a slush fund for God knows what. And Biden cancelled the Wall and keystone.
News (@KUSINews) Tweeted:
The federal government has reached an agreement to restore nearly $1 billion in funding for California’s troubled bullet train
https://t.co/GS1pfF8VXq
Yeah, there is something very weird here.
Nothing weird, just California-level grift.
Protecting their DEW / wildfire-starting “investment” along the route of this “bullet train” boondoggle of graft?
“Paradise” …
Yes ! They can burn up the whole corridor and ‘rebuild better’ on stolen property
Insane train from nowhere to nowhere, likely to be ridden by no-one.
Except maybe trafficking victims and coyotes…..
What, are we supposed to imagine a handful of windmills is powering that thing?
One of the more ridiculous ads the greenies put out was to show a nuclear power plant (and those are typically built to push out a billion joules per second which equals (anyone? anyone?) a billion watts. a gigawatt, 1000 megawatts.
Then wipe it off the picture and replace it with three bird killers. In fact you’d need thousands of bird killers to replace that nuke, and THAT is making the very stupid assumption they operate at full capacity all the time.
Its over a decade and costs going up and up. And nothing being done. Its wierd.
They can spend endless amounts of time “designing” the project.
I’ve seen highway projects here where the “design phase” cost as much as the actual move-dirt-and-pave phase.
All the grift for favored status.
Q: What’s BRIGHT fluorescent orange, and sleeps six?
A: A CalTrans truck…
But… but…. but… Always On Camera says they can put some on the top of the train, too, so it can be self-powered. Just add a few Unicorn Farts and Pixie Dust (remembering, of course, that the thing was pushed, if not started, by governor Moonbeam)…
We’ve got almost complete electrification over here, and the load on the net is enormous. Will be interesting to see what happens when all of our nukes are shut off, the Greens manage to shut off coal by 2030, and Uncle Vlad decides to turn off the spigot to punish Ukraine….
Maybe the days of Steam will come back 🙂 We have tons of locos preserved in museums (sad to say, nothing close to 4014 and its brethren, or even 3985, Challenger), but lots of serviceable “varnish”…
Oh, wait. The greens are all up in the trees, protesting….. Fridays for the Failure…
Oh, btw, thank God it’s Faraday (OK, a little late, but still :)… Nice article and series, especially since they’re about when science WAS about SCIENCE and not stuffing someone’s pockets. What was it Eisenhower warned about the Academic-Government Complex…..
“What, are we supposed to imagine a handful of windmills is powering that thing?”
__________
The windmills go on top of the trains, obviously. The faster the trains go, the more power the windmills produce, the faster the trains go, all the way up to light speed.
Which is the secret name of the project, it’s the Light Speed Train.
When completed, it will go from CA to NY in about 5 minutes.
4:59 to reach the speed of light, and then you’re there.
It’s an ambitious project, like all the best Leftist projects are.
The monkey wrench that’s holding everything up is how to slow the train down without overshooting the destination and going into orbit…
…of another star system… 👍 😁
ℕ𝔼𝕆ℕ ℝ𝔼𝕍𝕆𝕃𝕋
@NeonRevolt
3h
·
Conspiracy no more…
784 likes
28 comments
410 reposts
Is NeonRevolt back?
When I go to his Gab page, I still get the “This account is private. You must request to follow in order to view their page” message.
And the last article posted to his webpage was January 6th.
Yeah, Neon’s posting regularly on Gab again.
So does that mean I’ve been banned, if I can’t see anything besides the “This account is private. You must request to follow in order to view their page” message?
Half the fun of being banned is doing something to get banned — and I didn’t do anything yet!
Interesting. I wonder if you just got stuck in limbo.
I clicked the ‘follow’ button months ago, and it changed to “Requested”.
If I click it now, it goes back to saying “Follow”, and in the bottom left hand corner, it says “account unfollow success”.
If I click the green “Follow” button again, the follow button changes back to “Requested”, and in the bottom left hand corner is says “account follow success”.
But no matter what I do, I can see no posts at all, just “This account is private. You must request to follow in order to view their page.”
This is the web address I always used before, but isn’t working for me now:
https://gab.com/NeonRevolt
Same here.
This is pure speculation on my part, but it might be a matter of Neon needing to manually approve new followers or otherwise do something on his end. Try tweeting at him and asking him to approve your request?
Sadie, how do I do that?
As far as I can tell, he doesn’t have a Twitter account. When I looked for him there, I found a link (repost) from Gab, to ‘Great Awakening’, where one of Neon’s posts was re-posted, it’s pinned to the very top of the ‘Great Awakening’ group, if which it turns out I am a member:
https://gab.com/groups/2043
.
And I found another outside link to a direct post of Neon’s:
https://gab.com/NeonRevolt/posts/105376847328618279
.
In either case, I don’t see any way to send a direct message, but I’m not very familiar with Gab either. I mostly just used Gab to follow Neon’s page and post comments there occasionally.
What I meant was try posting a Gab starting with @NeonRevolt, or post a message in his Q group asking if he could approve your follow request.
Thank you!
I am seeing his posts occasionally but only as a re-post by who I assume must be one of his “approved” followers.
(if I stumble out of one I will post; maybe try “following” one of his “approved” followers ?)
EDIT :
That was easy !
It was re-posted by one of our very own:
https://gab.com/Bflyjesusgrl
😉
I get the same thing. But as I recall he blocked me for standing up for myself several months ago. And it was such an important exchange . . . that I can’t even recall what it was about. 😄 Just a little big for his britches I’d say. There are lots of smart people out there who can write, and are willing to.
We’ll find something 😆
Pre-emptive punishment – it’s all the rage, now 😀
(Brought to you and tested by the deep state, in Orwell’s UK)…
Oceania, ’tis of thee…
Kristi Noem on Fauci:
This uses the Gab TV embed builder, but the HTML code they give you does not stop it from autoplaying, even though it does not say that the autoplay is on.
THEREFORE, just copy the code with autoplay checked, and change the value from “on” to “off” after pasting it into the code popup here.
J+J Vaccine.
https://www.takimag.com/article/institutional-collapse/
“A year of outright lies from the scientific community regarding the origins and threat posed by Covid has severely weakened that trust. The scandalous efforts by the tech monopolies to suppress any debate over these topics have revealed their authoritarian instincts. The Fauci Affair is coloring all of this with the taint of foreign loyalty. To many Americans, Covid is looking like a year of ritual humiliation in service to foreign interests and private gain by connected insiders.”
“A year of outright lies from the scientific community regarding the origins and threat posed by Covid has severely weakened that trust.”
______________
Oh, here it is, the trust We the People have in the scientific ‘community’:
.
.
.
And what do we have here?
It looks like… yes, I think it is! The trust We the People have in the medical ‘community’!
NUKED!!!
Just look at that ‘trust’ go!
It blowed up real good!
From GAB:
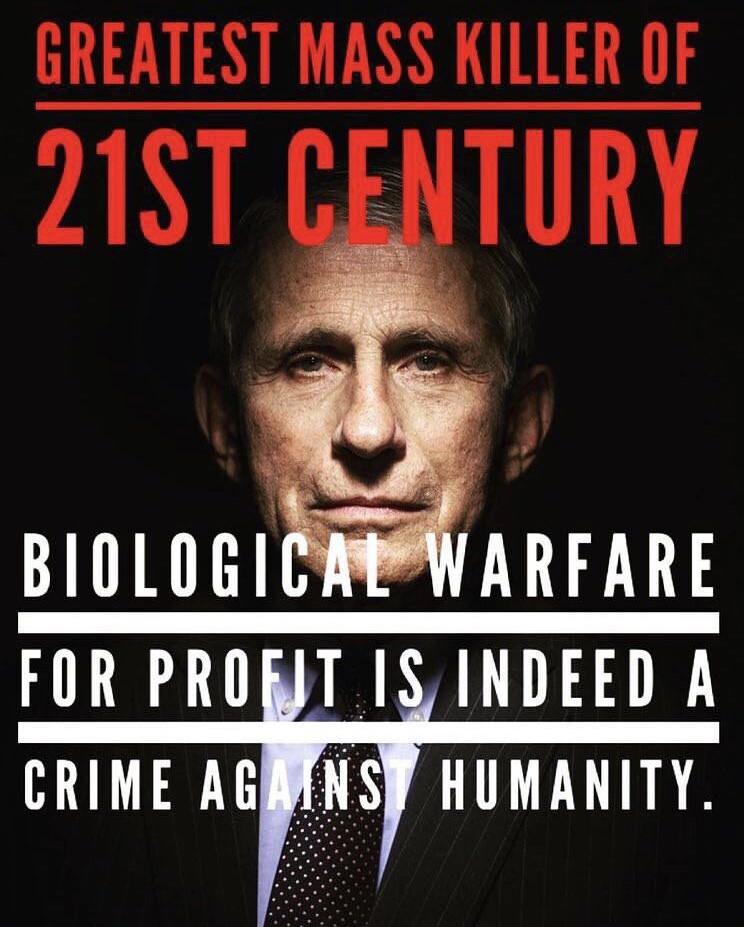
I’d imagine that they must know that there is an offsite copy of audit documentation and imaged data. And that if they showed up with a piece of paper to back this “seize everything” mandate, they’d be laughed at. And if they showed up with a full Federal assault force, there would be casualties on both sides.
Things would get really salty really fast if they went for option 1.
The reason the wicked Communist Feds are getting antsy is that they’ve been illegally spying on the audit, a la Holder, and they know what’s coming.
You bet they are!
And their spies are reporting back REAL BAD NEWS.
I really think that if they seize the materials in the AZ audit there will be deaths. I have never seen the level of anger in the country that I see right now. Small things are setting off explosions. It just happened here in my small town over a “pride” crosswalk proposed by some high school students. Shit went sideways FAST. The “quiet” Conservatives are not quiet anymore.
I hope the “Just-Us” Department has correctly assessed the risk here, and backs way the fuck down.
If you “Just-Us” guys are reading here, it is in your BEST INTEREST to allow this election to be corrected. Survive to cheat another day. I am saying this for your own good, just like your Mom used to do. And you know she was always right!
They are all in. PT will not let them fade into the sunset.
If justice does not happen they will regroup and be back in no time with even worse evil to implement.
Agreed. They always come back.
I think you’re right. They will use China AGAIN – maybe release a deadlier but still phony virus.
I don’t care.
The diamonds must be returned. And in a timely manner.
If not, the cops are crooked.
That needs remedy.
“It just happened here in my small town over a “pride” crosswalk proposed by some high school students. Shit went sideways FAST.”
___________
Good!
The brainwashed high school students promoting Marxism and Sodomania need to be confronted and SPANKED.
Almost certainly for the first time in their lives.
Oh, they’re getting spanked all right. I feel sorry for them. The adults in their lives have ill-served them, and now they are getting the lesson of a life-time.
Spanked? That lot might like it….. watch out for the spikes…😆
I remember those “Scared Straight” documentaries when I was a kid, where kids who were getting in trouble with the law would be taken to a state prison to see what life behind bars was like, and get confronted by real prisoners about how they were throwing their lives away.
Those ‘tough’ kids suddenly weren’t tough at all, when they were confronted by hardened criminals who were a lot bigger, stronger and tougher than the kids were.
They were intense shows. Really stripped all the excitement or allure out of being a ‘bad guy’.
A kid today would have to get PTSD counseling and crawl into a fetal position in a safe space for month if they saw one of those old shows.
Which is (one reason of many) why those shows probably couldn’t even be made today.
Not forgetting the immortal “Reefer Madness” 🕵👽😵
Sounds like another Waco in the making then to me. The end result my not be the same though.
Might be a larger version of what happened in Franfurt (FFM, Germany) with that server amid rumblings that Haspell was there and an interagency battle was going on…
Yellow tables have been gone all week. Red, blue, and green tables are dwindling, they’re on the last pallet. As the colors go, more and more white tables are erected. Looks like they’re re-imaging all the ballots right now. 😉
Don’t forget Waco. AGs have no problem violating state sovereignty on a imaginary pretense.
True, but that was after they picked a target, and froze it.
They can’t make We the People the ‘bad guy’, because it will only expose themselves as the real bad guys, for the whole world to see 👍😁
If they try Option #1, it should be a trap.
Surround the federal assault force and arrest them all, and put them on trial.
When the Feds send a bigger assault force, do the same, surround them with every law enforcement officer in AZ, plus at least a hundred thousand armed Arizonans.
Neighboring states go on full alert.
If the Feds show up with thousands, the People from every nearby state show up with hundreds of thousands.
If the Feds show up with a hundred thousand, the People show up with MILLIONS.
We the People ALWAYS win the numbers game, and they know it.
FEAR is the criminal government’s source of power.
We the People, UNITED and ARMED, have nothing to the fear from these third-rate bullies.
I’ve been a fan of the Prof’s advice after the meeting got raided in Moon is a Harsh Mistress. They should just disappear.
I haven’t read it!
You absolutely should.
It is a MUST READ.
Like huntin’ shinny up in the hills.
“Gee, chief — we went in and looked and didn’t find nothin’.”
I’m OK with either. I’m past anger.
I’m what might be called “resolved”.
The original post here is a beautiful exposition of how scientific discoveries in the late 19th Century started to froth over from one field to the next. Our understanding today stands on the shoulders of giants who literally took us from backwards phlogiston to almost modern chemistry over the course of a couple of decades.
I really wish that kids could get their first introduction to science with this perspective. Not, “everybody knows XXXXX” but “everybody ‘knew’ ZZZZZ, but weird stuff kept happening, and person YYYYY came up with an alternate explanation that made much more sense.” A History of Science with a bit more Kuhn than the old textbooks.
It was very well done, and my only regret is that it was too short…..but it really isn’t short. The presentation sweeps it along at quite a brisk pace.
In the interest of disclosure….my mom was going for a PhD in the History of Mathematics when I was in Junior High (she bailed), my parents have 5 STEM degrees between them, and I “cheated” in my own Econ undergrad by taking two “History of Science” courses for GE requirements that I probably could have taught (though not as well as Steve).
I ended up doing what many considered a 5-year program (Business Economics with emphasis in Accounting) in 3-1/2 years, graduating at 20. I’ve seen plenty of academic flim-flam. I consider Steve’s series so far to be excellent.
IOW, REAL SCIENCE was happening.
YES. But even then, the NEW YORK TIMES was pushing errors. I find that interesting.
😬
>>”a bit more Kuhn”<<
I take it that you’re referring to Thomas Kuhn, author of “The Structure of Scientific Revolutions”, published IIRC in the 60’s?
His point was that one mustn’t judge NEW theories / standards by OLD theories / standards. Doing so puts the cart before the horse, as it were …
In mathematics one never puts Descartes before De Horse.
Ha – very clever!
IMO, Descartes’ work has been quite under-appreciated by almost all subsequent mathematicians, even up until today, right now.
His famous dictum (from Meditations on First Philosophy [part VI, IIRC]) – while heralded as brilliant – means more than many know, or suspect.
Be sure to use the Euler (von Neumann) before Turing, or the friction might cause a Blaise before you Pascal (Ptolemy about that). But if you just keep it at a Cantor, Euclid be OK…
(sound of approaching hooves of GRAPHIC PUNishment, Drawn, Quartered, and indexed…). (What a Boole believes)…
Any statement that must be true by the rules of symbolic logic is a tautology.
Any statement that must be untrue by the rules of symbolic logic is Booleshit.
In the pre 17th century the term was “natural philosopher” and the impression I have gotten they were the ones who dealt with, basically, what we think of now as physics, chemistry, and astronomy. There weren’t any borders between those subjects, and as we will presently see it’s amazing how connected the very small is to the very big. Of course, they weren’t doing actual science, but speculating very learnedly. (They didn’t test their speculations, though they did usually try to be consistent with what they saw around them. They didn’t recognize the need to set up new situations to see if they, too, would conform with the theory.)
The terms natural philosophy and natural philosopher stuck around at least to the end of the 1700s, and still gets some use today.
And I positively agree about how to introduce to science.
I was one of those kids and got a good dose of science in books I read as a kid; most others first had it inflicted on them in 6th grade, and it was taught as a string of “units” unconnected with each other, which simply asserted things. Correct things, as it happened, but still, just bare assertions which some pseudoscientist douchebag can just call into question later. With no understanding of why we know things to be true it’s easy to be persuaded they are not by some reasonable-sounding argument with a lot of big words in it.
The interconnections and the history are vital. If someone tries to claim “quantum mechanics is complete bullshit” and you know that much of modern life is actually based on our understanding of it and the very thing you’re reading these sentences on couldn’t work if quantum mechanics weren’t true…you will know to laugh at the guy. (We argue over the interpretation, but not over whether it happens the way described.) You know just how solid the evidence is that the universe works that way because it’s not just some stuff on a blackboard somewhere.
Yup. The knowledge of REAL SCIENCE was fought over by people who CARED ABOUT TRUTH.
The Soviet Union and China “colonized” our government as a commie power via the DNC.
Of course it’s hostile.
Like a Soviet satellite state during the Cold War.
Except we have a LOTTA guns.
Well over half a billion at last count.
A hostile government…our own hostile government.
Summarizes it perfectly! He was right…just not in the way he was thinking.
Bidung’s got it backwards: Biden(s) (the crock and the “Doc”) are the greatest threat to US and indeed, the West’s security.
Climate change PROPAGANDA is definitely a threat – just like critical race theory – and both of them are MADE IN CHINA and repackaged as “domestic” here!
And it’s not even new… it’s more like a Revival… 😂 🤣 😂
If he shuts down AZ, it will light all the others on fire and draw huge attention to them.
Then they will have to shut those down too, under the white hot spotlight of the entire world.
Go ahead, Merrick.
Make my day.
Light the fuse, Merrick.
We’ve been waiting a long time for this.
On second thought, how are the Feds going to shut ANYTHING down in AZ, when the Senator just said something to the effect of putting the AG in prison and the court just ruled that the Sheriffs are the highest Law Enforcement power in any state, above the Feds…
Do it, Merrick…
I wanna see you try…
Go ahead.
Everyone is calling your bluff.
And when you are forced to back down the first time, your faux invincibility is SHATTERED, and it’s all over.
Do it. Do it. DO IT!!!
😁
Back at the university, at one point, when I asked the advisor for permission to take a history class pass/fail instead of a letter grade, he remarked: «History? That’s important!»
I did so well in that class that I might have got an A instead of just Pass, but ever since, that remark has never been forgotten. And the older I get the more I realize that he was right. So should anyone ask me now, I’ll reply the same.
I’m really enjoying these excellent historical physics lectures about the state of the art as of 1895, as well as the giants on whose shoulders we now stand. I see plot lines moving forward, but I’m not telling…
Yes.
People have been very, very good about not posting spoilers, and I appreciate that.
It will soon become apparent how much I’ve had to tie myself in knots not using some words and concepts we take utterly for granted today.
To your original statement. Robert A. Heinlein considered three subjects vital. Languages, mathematics, and history. He actually once said that if you didn’t know a lot of those three you were an ignorant peasant with dung on your boots. A lot of the characters in his books were a bit more parochial, like the father who didn’t care that his identical twin sons had cheated in both history and math by having the same twin attend both history classes and the other twin attend both math classes. He wasn’t worried about the history class but he made the twin who hadn’t attended the math class go learn it at home.
Verse of the Day for Saturday, June 12, 2021
✟
“…for this day is holy unto our Lord: neither be ye sorry; for the joy of the LORD is your strength.”
Nehemiah 8:10 (KJV)
Thank You, Jesus, for blessings received and prayers answered!!!
BE MY VOICE
PRAYING ON THE ARMOR OF GOD
Father God, I now follow your command to put on the full armor of God, because my battle is not against flesh and blood but against rulers, authorities, the powers of this dark world and against spiritual forces of evil in the unseen world.
I first pray on the Belt of Truth that it may be buckled around my waist, may I be centered and encircled by your truth dear Lord. Hem me inside all that is true and right, and may I be protected and held up by the truth of your living word, in my Lord Jesus name.
I pray on the Breastplate of righteousness, please protect my vital organs and my inner man, cover my integrity, my spirit, and my soul. Guard my heart for it is the wellspring of life, please strengthen and guard the most vulnerable places in my life with that which is right, good, and noble that I might not receive a fatal blow from the enemy, in my Lord Jesus name.
I pray on the Gospel Shoes of Peace. I choose to stand in the shoes of your good news, and on the firm foundation of my Lord and Savior Jesus Christ, the solid eternal rock. All other ground is sinking sand, I pray that I will not slip or fall, but that my feet would be firmly fitted on your lordship, my Lord Jesus. I choose to stand on you, so that the peace of God, which transcends all understanding will guard my heart and mind in Christ Jesus, the eternal Rock of Ages. I receive your holy peace now my Lord, from the sole of my feet to the crown of my head, in my Lord Jesus name.
I pray the Shield of Faith into my hand now. As I take up the shield of faith, I ask that you might extinguish every dart and arrow, that is launched from the enemy to take me down spiritually, physically, mentally, emotionally, and every attempt of the enemy to destroy my joy. I ask that my faith in you would make it flame out. Extinguish every flaming arrow that would come against me, my life, my family, my home, or my ministry. May my faith always be out in front of me like a shield. Give me the courage to “faith my fears” by choosing to walk by faith and not by sight, in my Lord Jesus name.
I pray on the Helmet of Salvation, that you might protect my mind from the thoughts that can lead me astray. I choose to take every thought captive, and arrest all intentioned ideas and motives that would harm others, or distract me from your holy will for me. I submit every captured thought to the Lordship of my Lord Jesus Christ, and ask that you would imprison those thoughts that are not of you my Lord. Transform my mind and renew my thinking that I may think God thoughts, and have a sober mind that is focused on your glory. Please protect me from being double minded that I may allow my mind, I reject to live an earthly life, because I choose to live a holy one, governed by you My Lord Jesus, the prince of peace, please have my mind to be saturated with the holy mind of Christ, in my Lord Jesus name.
Finally, I take up the Sword of the Spirit which is the holy word of God, I pray this powerful offensive weapon into my hand, and ask that your holy word would be fitting for every encounter I face. As the enemy gets close to me, please give me the insight, wisdom, and skill to wield the word of God to drive away the enemy, in my Lord Jesus name.
May the enemy and his team flee from me, upon hearing the word of God spoken by the power and direction of the Holy Spirit. Give me the sword of the spirit to cut through the wiles of the devil, so that I may discern the schemes of the enemy when he is near.
With all kinds of prayers, supplication, and intercession I pray to you my Lord God as the one who fights my battles. Now that I’m in your holy powerful armor, I walk away covered and ready to face my day as you go before me, and please protect me in the midst of the spiritual warfare in this unseen world, in my Lord Jesus name.
Thank you my Lord, for the spiritual weapons of armor and prayer that you have given me. It is written no weapon formed against me shall prosper, and you will refute every tongue that accuses me.
Thank you Father God, my Lord Jesus and the Holy Spirit, that I am more than a conqueror in my Lord Jesus. I pray all of this in the mighty name of my Lord God and Savior Jesus Christ, amen.
Well, this is ** interesting ** in the least —
It appears that the VAERS (Vaccine Adverse Event Reporting System), https://vaers.hhs.gov/, is no longer accessible online by the general public (maybe MD’s can still get in to report). Error message of “Hmmm….Can’t reach this page”
“Suspicious dog” all over this one. One wouldn’t be surprised if Becerra ordered VAERS to close down access to the general public. One wonders why.
Very interesting.
https://twitter.com/Littleb29872980/status/1403632116139802625
Holy shit. It won’t even load on the Wayback Machine.
Error message comes up:
Fail with status: 500 Internal Server Error
I found the way in – we have to go through CDC now. They monitor who’s in there, and you have to agree to terms!
https://wonder.cdc.gov/vaers.html
Interesting. I just tried the regular way in again and it worked.
I wonder what they were purging/coving up/changing so we couldn’t find it?
Yup – working for me now, too.
Sundance just put out a new article about the number of deaths attributed to the injections.
https://theconservativetreehouse.com/blog/2021/06/12/cdc-vaccine-adverse-event-reporting-system-vaers-shows-stunning-increase-in-deaths-due-to-covid-vaccine/
It makes me wonder…
It appears that the U’s are doing a lot better than the rest.
Must be a new ‘protected’ class… 😁
Naah, maybe there were only 203 of them in the US.
You can be certain that Merrick Holder has that whole bunch spying on all of us, including Sundance. Third Osatan term will be continuing all their tricks.
This sentence is ambiguous. Is Sundance one of the spy-ees or one of the spy-ers?
One of the “spied upon”. That was the Holder pattern (no pun intended). They kept tabs on “opposition journalists” to get ahead of any narrative.
Yeah, they MOVED IT. Access is no longer through HHS. You have to go through the corrupt and lying CDC. And they’ve made it complicated as hell.
Remember how Trump had to move stuff OUT of the CONTAGION – the INFESTATION in CDC? How he moved much reporting to HHS, and we finally got good COVID numbers? They must have moved access to VAERS there as well.
NOW you have to GO THROUGH CDC.
Here is the link, which I had to pass through MULTIPLE web pages to find. They REALLY obscured it.
https://wonder.cdc.gov/vaers.html
Here were the links I followed:
https://www.cdc.gov/coronavirus/2019-ncov/vaccines/safety/vaers.html
https://www.cdc.gov/vaccinesafety/ensuringsafety/monitoring/vaers/index.html
https://www.cdc.gov/vaccinesafety/ensuringsafety/monitoring/vaers/access-VAERS-data.html
https://wonder.cdc.gov/vaers.html
Wolf Moon
HHS and CDC both panicked now that the truth about the “vaccines” is being published, perhaps?
You can search the VAERS db through this link (and it’s working) although the data is only until June 04, 2021:
https://medalerts.org/vaersdb/index.php
Thank you! A nice link – very straightforward to search.
Thank you, Wolf.
https://jamanetwork.com/journals/jama/fullarticle/2778441
April 5, 2021
JAMA Insights
“Reactogenicity Following Receipt of mRNA-Based COVID-19 Vaccines”
https://jamanetwork.com/journals/jamapsychiatry/fullarticle/2778090
March 26, 2021
“How COVID-19 Affects the Brain”
Greater tendency of Moderna to produce side effects relative to Pfizer, observed in clinical trials, was CONFIRMED in actual use.
Hey Smiley, Where are you?
Hope you are well, busy doing art and fun stuff – enjoying our great Governor!
There’s something in the Gulf!
I’m missing T3 too!
And wheatie!
Oh my gosh! I’ve been stuck in my daughter’s wheelchair and apartment and health issues stuff too long and don’t know what’s what. Is Wheatie ill or going through a tough time?
I haven’t seen her here in the last few days, so hope everything is ok.
Many awol. Dunno where they all are. Even a pop in now and then would be nice from a lot of long timers. I thought its election plus busy spring but people are gone. Pat Frederick doesnt visit and phoenixrising left too.
I’m advising everybody to get break time and preparations in BEFORE things get “awe-inspiring”.
Nothing wrong with that.
It’s hard to get that MAGA mojo rising when things are so damn convoluted. There’s so many issues…some seem impossible to overcome, others are one step forward one step back…some seem on the verge of victory and then…wahwahwah more issues.
We were traveling across the country to S Carolina. I’m having a hard time adjusting to the 3 hr time diff and there’s a lot to do here. I did get a small garden planted. LOL it would feed us about 2 meals in about 3 months! Gas is so much cheaper here. No masks except a few diehards here and there.
Yeah totally different from here.
Btw major heatwave starting tomorrow. Going to 108 on tuesday, over 100 the rest of the week. Not fun!
whoa! Did you get the pool you were talking about before??
Yes finally. Its a cheapy $59 pool but deep enough and wide enough to be worth calling a pool. Teeny pump and we chlorinate it.
No june gloom! Way way too early to get this hot.
You may want to throw a shade over part of it.
Its sort of got that bc of where its situated. But no going out without our sunscreen.
PS` Huge thanks to all who bring the sauce everyday. I think a lot of people are reading and soaking up the facts even if we aren’t posting as much
Thanks for your Saturday Posts, Mr. Steve! That eagle matches my mood post election!
You’re welcome!
Someone mentioned VAERS being offline. Maybe this is the reason:
SHOCKING JUMP in Vaccine Deaths Reported This Week at CDC-Linked VAERS Tracking Website
https://www.thegatewaypundit.com/2021/06/shocking-jump-vaccine-deaths-reported-week-cdc-linked-vaers-tracking-website/
Deaths are now at 5.997.
I believe this is due to them shutting down the easy HHS link.
Here is the link through CDC.
https://wonder.cdc.gov/vaers.html
New Yorkers will change montana like a lot of east coasters changed CA. Not as fast maybe as CA bc we had all the illegals too, but its a warning .
Make L.A Great Again
– RECALLGAVIN2020.COM (@lalovestrump) Tweeted:
Montana, the sold-out state New Yorkers can’t get enough of https://t.co/9HFcrJMy5f via @nypost
I really don’t think most of them will stay.
It’s really cool to live in Montana for a while, then reality hits. It’s cold as hell for six months, mostly cold for a couple more, then hot as Hades for three months. Extreme. And sometimes the temperature can swing 60 degrees in a day.
There’s not much to do, not much night-life, only a handful of fancy restaurants, no theater scene to speak of. Nobody makes any money, not even attorneys and doctors. At least not like they do in bigger places.
Montana is still farm and ranch country with a few small cities thrown in. Big city people get bored and leave. We don’t mind the vacationers and part-timers; they leave lots of money when they go.
One really bad winter will take care of most of the ones who thought they’d stay.
If they do what happened in socal….developers come in, buy everything, sttip malls, furniture stores, massive # of homes, apts, etc.
I hope youre right tho.
Mr gils friend who left wanted the lifestyle and they dont even want to visit in CA.
Wonder if he swiped the scarf from Birx?
To the tune of “Kung Fu Fighting”:
Oh-hoh-hoh-hoax
You stooped so low
Oh-hoh-hoh-hoax
You’ve gotta go…
[Chorus:]
Everybody was Kung Flu Fighting
Those docs were fast as lightning
In fact, it was a little bit frightening
But they fought with experts lying
There were funky China men from funky Chinatown
They were faking them up
They were vaxxing them down
It’s an ancient Chinese art
And everybody knew their part
From lying through your lips
To a needle in your hip
Everybody was Kung Flu fighting
Those docs should be in hiding
In fact it was a little bit frightening
But we’ve caught the experts lying.
There was funky Dwarf Faux-Xi and little Deborah Birx
He said, here comes the big boss, let’s see if this works
We took the spikes and made a shot
If it don’t work, then man it’s hot…
A lawyer’s motion made me skip
Now we’re gonna’ have to take a trip
Everybody was Kung Flu fighting
Those docs were fast at lying
They made it seem so frightening
Fake numbers went up like lightning
Oh-hoh-hoh-hoax, ha
You stooped so low, ha
Oh-hoh-hoh-hoax, ha
Keep on, you’re gone, you know
You’re gone, sure enough
Everybody was Kung Flu fighting
Evil perps should be struck by lightning
It had better be more than frightening
You know you had experts lying
Kung Flu fighting, you’ve got people dying……
[Apologies to Carl Douglas, and everyone else in the known and unknown universe… with the exception of the Kung Flu “team”…]
Matthew Shepard vs. Jesse Dirkhising. The media is a RELIGION HATE ZONE.
Great whites are amazing fish but i dont ever want to be this close to one! Leopard sharks will be in san diego to mate and have babies soon. Thats more my speed.
“Professional shark taggers warn there are at least four great whites lurking off Long Island and New Jersey en route to Cape Cod – with a 600-pounder named Rose and a monster 16-foot, 3,456-pounder called Mary Lee right behind them.
…
As of Friday, tagged sharks in the New York area included Charlotte, an 8-foot, 300-pound juvenile; Martha, a 7- foot, 184-pound juvenile, and Monomoy, a 6-foot, 7-inch juvenile male. Rose, a 10-foot, 5-inch, 600 pound behemoth, is expected to arrive this weekend from Delaware Bay; and Mary Lee, a 16-foot, 3,456-pound monster is lurking off Long Beach Island in New Jersey.
Andromache, a monster 11-foot great white, made a stop in local waters last month and was last detected summering off the coast of Cape Cod. Among the largest sharks Osearch has tagged is Nukumi, a colossal 17.2-foot female great white, who recently returned to Nova Scotia waters after a deep dive swim over the mid-Atlantic ridge.”
https://nypost.com/2021/06/12/huge-great-white-sharks-lurking-off-ny-and-cape-cod-coasts/amp/?__twitter_impression=true
Unfortunately, I seem to hear that whisper even without the whiskey……:0)
One of the benefits of not being house broken, usually speak my mind.
Surely, nobody here needs that sort of reminder…..
Have to, otherwise somebody will steal it! 😁
New way to foil cattle rustlers — leave the cow in the back seat of a parked car…..with a stick shift!
I guess the original working title Wooden Nickel was rejected then?
In favor of “metallically challenged wafers”
I am seeing lots of notes that hollywierd stars are moving to Australia.
What is going on in the last year that this is a thing? Seriously big names. Proximity to China? Cabal hq? What is it.
Claims Julia Roberts is ‘pulling some strings’ to get her children enrolled into exclusive Catholic schools in Sydney’s affluent North Shore
https://www.dailymail.co.uk/tvshowbiz/article-9679525/Julia-Roberts-pulling-strings-kids-Catholic-schools-Sydneys-North-Shore.html#article-9679525
Proximity to their New Zealand bunkers. I guess I’ll throw Antarctica in there, too, since we’ve had at least one headline about Tom Hanks visiting there.
There was a film called Greenland that came out recently…
I liked Gerard Butler but every other movie is an apocalyptic climate change story. Hes done like 3 or 4 now.
Great. Hope all the hollyweird fools leave, soon.
Not speculating, but I will. Danish player collapses during game. CPR required. Vax related I bet .
https://twitter.com/disclosetv/status/1403759772818677760?s=19
If he had WPW or a hidden arrythmia it could get ugly… hope and pray he’s OK…
They paused the match for 1.5 hours, but the players asked for it to be resumed. The player who collapsed, Christian Eriksen, was reanimated and is currently in the hospital recovering, and they are cautiously optimistic about his condition. Some players spoke to him, so he is conscious… would be curious to know what the real situation is, because folks that young (and in that good condition) have an extremely high survival rate.
For athletes with inborn heart conditions, if can get difficult, especially with some that are “hidden”. We know a fellow who had Bradycardia, where his heart beat too slowly. Eventually it got so slow he collapsed on the field, and ended up with a pacemaker.
(minor note, this kid LOVED getting jabs, all the blood tests, infusions, taps, you name it.. but he soldiered through it all)…
It was the jab.
OMG.
Why??? Who put him up to it (or WHO put him up to it)…
It’s not the World Cup, but it’s the European Championship, so a TON of people will have seen it.
Don’t think they can push this aside…
Still, I hope and pray the player is OK.
Plays for Inter Milan and the team doc confirmed all players were vaxed end of May. Latest is he is good.
100% unrelated to the injection, which IS 100% safe.
Ask FauXi.
If nothing else, the benfits out weigh the risk. Just ask, Christian Eriksen, the player. Ask his wife, kids…
(sarc)
That was my very first thought!
International level players just don’t collapse. If they had a bad ticker would have been found out years ago.
Not in the case of concealed WPW. There have been SCDs (sudden cardiac deaths) from athletes in different sports, not just football (soccer) that have had this. Lack of proper testing for athletes at many levels is a controvery, at least over here. Funny (not) how there’s plenty of money for Wu-Flu tests, though, and the jabs at 3K€ each…
I know, but it is extremely rare. You don’t get to that level with a bad ticker.
I posted some papers on the thread for the 13th. There have been people at that level who’ve died. Look up “concealed WPW” in Wiki, pub med, or Google Scholar.
My son had concealed WPW, and I’ve probably spoken with many, MANY cardiologists of various levels and specialities over the last 10 years, and the hidden arrythmias are one of the toughest nuts to crack, especially if the ones at risk who should be tested, aren’t. I was watching the “replay” of an EKG after one “episode” when he was in the ICU, and, to that point, they were strenuously fighting the possibility that my son’s problem was WPW (despite it being sprinkled among the family). There were seven cardios sitting there, including the top one. They run the “tape” backwards and forwards, and then one of them yells “That’s it”. They enlarge the segment, and, sure enough, WPW.
Sometimes they get preconceptions in their heads, e.g. gotta make everything into a nice syndrome package with a bow on it, and refuse to see anything else. The paper I quoted on the 13th post has outbound links which show examples of this and other problems.
My son’s “rommate” in the ICU, for instance, had hidden Bradycardia and collapsed on his practice field. Fortunately someone was there to give CPR (they had no DeFi) and he got a pacemaker. Very well-trained athletes are usually right at the edge of Brady, because they have a very low resting pulse, but they also have enlarged hearts, which puts them at risk for arrythmias, in particular the hidden kind I mentioned.
One of the doctors wryly observed that the pacemaker needed cost around as much as a new Mercedes. But it did a lot more good!
General McInerney
Eric Swalwell’s phone was subpoenaed by Trump Administration. Of course he’s a spy…we have it all.
Eilert, NOT intended for you… More likely McInerney and blathering fools like him creating click bait. Yea, they gotta make a living like the rest of us.
—-
Uh, OK. Phone was subpoenaed. Sounds GREAT! Swalwell should be in deep shit!
Wait. So DoJ or FBI have Swalwell’s phone?
Aren’t these the same asshoes that set up Trump, Trump officials, trump family, Trump friends…?
Same asshoes that have NEVER prosecuted anyone in the Russia, Russia, Russia hoax OR any of the witch hunts against Trump?
FISA abuses? Skip that one, FISA abuses. Wray held more training, in how to cover up FISA abuses. We won’t see any more of those. Dumb asses in FBI have training in pencil whipping forms to look legit.
Same DoJ and FBI shit heads that ignored hunter’s laptop and other evidence he is a felon waiting to be chargd accordingly. Ukraine, drugs, lying on forms to buy a gun…
The same DoJ and FBI that have ignored the stolen election? NOW these shit birds are interested in interfering in the Arizona audit.
BY the way, where the hell is “Bull Durham”?
DoJ and FBI creating criminals of of simple white folks…allegedly white supremacist terrorists.
Now, if John Solomon or Sarah Carter report this, we can also
—
CLEARLY, this F O O L is unimpressed with McInerney’s blathering.
Close enough for government work?
https://www.tigerdroppings.com/rant/politics/til-the-commie-cadet-is-now-a-professor-at-u-texas/96783785/
Commie asshat.. quote~
Spenser studies modern Arab intellectual history. He is broadly interested in Arab thought as it pertains to decolonization, political metaphysics, consciousness, subjectivity, and temporality in the twentieth century Arab Middle East. His research is primarily concerned with the question of the spirit and soul in modern thought. Specifically, he seeks to reconstruct and historically situate politico-theological ideologies such as Ba?thism and similar thought-forms in the Arab World and beyond.
Laughable.
Well, let’s see: Baathism wants to “unite” the key elements of both Islam and socialism (Communism) into one seamless method of command and control.
The word salad about this “professor” doing research on “the question of the spirit and soul in modern thought” is “grant-application” speak.
Not to put too fine a point on it, he’s working on how to make Islam + socialism more “accessible” / “acceptable” in the West — and specifically, in the United States.
Nothing like working on ignorant college kids to get this seed planted.
Right up The Squad’s alley.
…Arab intellectual history…
^^^ Can’t stop laughing. ^^^
modern “…Arab intellectual history…”
Car bombs, blades and rockets.
Before that it was just car bombs and blades.
And then there’s ancient Arab intellectual history, which is more commonly known as just the history of blades.
It’s not the Arab that is the problem, it’s the Mohamad virus.
There have been Arab renaissances, and so on, but then some fundie Islamic nut takes over and squelches it.
And I think rockets predate car bombs, but your point was clear!
“Spenser studies modern Arab intellectual history. He is broadly interested in Arab thought as it pertains to decolonization, political metaphysics, consciousness, subjectivity, and temporality in the twentieth century Arab Middle East.”
_____________
So he’s studying how to murder all of those things?
Modern Arab history is the same as ancient Arab history, in that everything revolves around murder in the name of Mohamad.
How is that even possible? How does he pass the background check?
The same way they vetted Zero’s would be my guess.
Don’t burn Wolf’s Tree down, folks!!!
Maybe some ISPs are down again?
AWS has been REAL wobbly over here because of infrastructure issues… somehow El Reg managed to stay online… (must’ve been the BOFH what dunnit)…
Some nutjob (MKULTRA victim?) tried to hijack an American plane.
Last one appox 8 days ago, LAX Delta386 to Nashville, diverted to OKC. Stopped by 2 air marshals.
https://www.laweekly.com/fbi-investigating-attempted-hijacking-of-flight-from-lax-to-nashville/
This one is LAX Delta1730 to Atlanta. Diverted to OKC. Stopped by passengers.
This is the kind of stuff that will bring wider exposer to MKULTRA, responsible or not. 😎
Totally futile. They can’t breach the door and the passengers will take care of business. The only question will be how many cuts and bruises he’ll end up with.
But it gets eyes off the Senate presser.
Ok ty. I thought it was the same nut but 2 now. Interesting. Both black males too.
That’s what I was thinking, recycled news story, but apparently not.
My wife, flight attendant, says there have been five in the past few weeks.
Wow. Wonder what the deal is? Just tired of the lockdown regulations?
Could be. Strange either way. A lot of airlines not offering much service also to reduce passenger contact with crew but that is loosening up.
Sounds like the Left is trying to create a false flag terrorist incident.
https://twitter.com/4_04_Not_Found/status/1403795563699712016
Excellent. US Senate is catching up with Deplorables and finally joining the fight. The wall of deceit is being ripped apart! 😜
Will the MSM now do their job? (Doubt it, but it just won’t matter anymore. 🙃 )
This has to be freaking out the G7. 😮
Now do the freaking election! 😡
Matt Taibbi and Glenn Greenwald are absolutely destroying the Pulse Nightclub narrative on Twitter. Of course, they don’t talk about the part where the whole thing was a false flag, how the shooter’s father showed up on stage with Hillary Clinton two months later, how the shooter’s wife disappeared for awhile after the shooting, and so on, but baby red pills are better than nothing.
It almost seems like the Fake News is trying to cut a deal by becoming more honest about things. They’re BARGAINING.
Even well known liars tell the truth on occasion. And that NBC article was published in 2018, not in the last few days. Just sayin’
“They’re BARGAINING.”
_________
Reee-jected!
😂🤣😂
Hey, folks! Be the first on your block to get in on this one!
http://www.msn.com/
Credit to Politico
1 hour ago
“Fed explores ‘once in a century’ bid to remake the U.S. dollar”
Down and Dirty Summary:
The U.S. Federal Reserve is beginning the preliminary moves to launch ITS OWN VIRTUAL CURRENCY. The move comes after recent “bi-partisan interest” on the subject from Senators Elizabeth Warren (D) and John Kennedy (R). It’s being touted as a way for “disadvantaged” people who don’t have bank accounts to “participate more” in the U.S. economy. The American Bankers Association is against any move in the new direction, since any potential virtual U.S. currency would operate from an individual’s “virtual wallet”.
The Federal Reserve Bank of Boston and MIT’s Digital Currency Initiative will publish the first stage of the their research on the new currency next month to determine whether or not it would work “on a practical level.”
One “advantage” of a Federal Reserve virtual currency, according to the parties pushing it, is that this would “drive out bogus virtual currencies” and “create more inclusion” for “disadvantaged” people in the U.S.
There are more details in the linked article; this is a short summary. However, what can be deduced from the above are:
The fact that the Federal Reserve and the MIT “wizards” have been working on this concept for some time, in order for them to publish a report next month. Who gave the go-ahead for this? Who paid for it? Who are the people involved?
What’s going on with Sen. John Kennedy, a Louisiana Republican?
It’s clear there’s an attitude that “privileged white people” in the U.S. kept “disadvantaged” people from having and using bank accounts — so the bank accounts of the “privileged white people” now have to be annihilated in favor of a virtual currency directly tied to the Federal Reserve (those nice people who print fiat money day and night, you know).
Anybody who doesn’t realize that a Federal Reserve-based virtual currency will be used as a spy tool on the entire American population, is living in an alternate reality.
This scheme could well be the first step in the permanent outlawing of owning precious metals by private individuals (think FDR’s 1933 gold confiscation EO).
More than just a spy tool. A tool of Oppression. What you like more, eating or your guns? Choose, cause your account is now frozen. I’m sorry, our digital dollar can not be spent on that, or this, or that, including those, and these. No to Cashless Society!
And get that bum Kennedy out of office. Haven’t liked him since he heckled Laura Loomer with that auction call.
And then there’s Black Rock turning us all into serfs again through SFRs.
(Single Family Rentals). They are all about the Great Reset…back to feudal times. What dirty POS!
https://thelibertydaily.com/yes-blackrocks-home-buying-spree-should-concern-you/
Disappointed in John Kennedy. He should know better.
Apparently the Senate Repubs are averaging about a year lag time in understanding what their constituents know thus he may not be aware yet he’s
loosinglost cred.VERY important piece for accurate perspectives….
https://theconservativetreehouse.com/blog/2021/06/12/mike-brown-sr-joins-group-demanding-to-know-where-tens-of-millions-of-donations-to-blm-have-been-spent/#more-212910
Michael Brown Sr, the father of Mike Brown from Ferguson Missouri, has joined a group of activists demanding to know where all the millions of donations to Black Lives Matter have gone.
They paid it to biden and took their cut.
It was discovered way early.
Anyone complaining now is single digit iq.
Anyone complaining now is single digit iq.
^^^ Racist! ^^^
That’s the white spelling.
They spellz it this way now…
^^^ Rayciss! ^^^
👍😁
https://youtu.be/kMGj_izIH9s
https://twitter.com/Johnheretohelp/status/1403740325550645249?s=20
https://twitter.com/Johnheretohelp/status/1403740333503025152?s=20
https://twitter.com/Johnheretohelp/status/1403749014315716610?s=20
https://twitter.com/Johnheretohelp/status/1403767614896279554?s=20
hteat exhaustion from maga work ouside all day
very sick and trying to keep something down
pleases pray
i will be ok but auful to go thorugh + all other health issues
almost asking to go to er for iv but rying not too
🙏 for immediate relief.
In case your too confused to look for a link…
https://www.mayoclinic.org/diseases-conditions/heat-exhaustion/diagnosis-treatment/drc-20373253
^^^ Great information at the link. ^^^
IF you think you need the IV, go for the IV. Wondering if urgent Care does IVs.
Too late now. But H Y D R A T I O N is ALWAYS Priority One.
(I learned the hard way.)
They can but dont like it. Basic nirmal saline iv but its the monitoring they don’t want to deal with. Shes going to have to be there couple hrs.
Really she can drink a liter or 2 of pedialyte, no food, and see if that helps.
Way better than Gatorade .
Yea. What little I’ve seen of urgent care is not impressive. Minimal time with the quack. Minimal observation.
Sorta like a “get’m in and get’m out”.
The cheapest (and just as effective) way to do it is to mix up a bunch of ORT. Pedialyte also works (and can be had in little packets, like kool-aid), as well as Electrolit.
ORT [ https://en.wikipedia.org/wiki/Oral_rehydration_therapy ] can be mixed at home from common ingredients. There are a variety of recipes — the official WHO one is one liter of water; 2.6 grams of table salt; 1.5 grams of no-salt (KCl); vitamin C, in the form of 2.9g of Trisodium Citrate Dihydrate; and sugar, in the form of 13.5g of anhydrous glucose. For home use, I’d recommend 14g of whatever sugar you have laying around (except HFCS), and add lemon or lime juice to taste.
The sugar is important, because your body will prioritize the absorption of water containing it.
When youre sick, and convenience and sterility is an issue, i prefer bottled. But youre right, thats the cheapest and still effective route.
A Summer heat dehydration public service reminder:
Drink 2 qts of water daily .
Sending positive thoughts your way.
If this has happened to you more than once, you should keep a liter of ORT in your car.
https://theconservativetreehouse.com/blog/2021/06/12/cdc-vaccine-adverse-event-reporting-system-vaers-shows-stunning-increase-in-deaths-due-to-covid-vaccine/
Up to 6,000 deaths…
Whoa!!! O. M. G!
Don’t normally link Twitchy, but….
https://twitchy.com/brettt-3136/2021/06/12/this-wasnt-well-thought-out-prides-tweet-sending-out-lots-of-pride-looks-kind-of-iranian/
Let’s see if this works:
Doesn’t this seem a little bit like throwing gays from the tops of buildings?
ARKANCIDE ALERT. The reporter who broke the secret Clinton-Lynch tarmac meeting story was found dead from “suicide.” He wrote an entire book about it.
https://www.al.com/news/2021/06/christopher-sign-birmingham-tv-anchor-former-alabama-football-player-dead-in-apparent-suicide.html
https://www.foxnews.com/media/loretta-lynch-bill-clinton-tarmac-meeting-new-book
https://www.washingtonexaminer.com/news/reporter-who-broke-story-on-clinton-lynch-tarmac-meeting-theres-so-much-that-doesnt-add-up
Wow. They are vindictive. Criminey it was years ago.
It’s as much about sending a message to other journalists and whistleblowers as it is about revenge. Especially with all of the looming election audits and COVID shenanigans.
I wrote something similar before seeing your post. Definitely a warning! It’s going to get more dangerous from now on. I hope people like Mike Lindell, Patrick Byrne, Mike Flynn, Steve Bannon, and many others, including local Arizona audit people, have good security.
It’s always been this dangerous — it has seldom been this exposed.
It should have exactly the OPPOSITE effect.
It should make more people come forward, safety in numbers, because they can’t murder everybody, and the more people they do kill, the more obvious it becomes who is really responsible for all of the murders.
When normies start referencing the Clinton Body Count list, it’s all over.
Returned to alabama to be an evening anchor, 3 kids, married. But killed himself. No details either.
Slick Willy and hildabeast NEVER forget.
I am really sorry to see this. Apart from the sad facts, it serves as a warning to those who would fight a certain political machine.
CodeMonkeyZ https://t.me/s/codemonkeyz
Is Stephen “Chucky” Richer from the Maricopa County Recorder’s Office blocking the auditors from seeing the SPLUNK LOGS?
Something to hide?
Caught red handed?
Who will crack first?
CodeMonkeyZ
Dont forget about ELK and grafana.
1
CodeMonkeyZ
Fraud vitiates everything.
CodeMonkeyZ
Did your meeting with the printers go well this week?
Panic.
CodeMonkeyZ
Think you were being sneaky?
Meeting caught on tape.
Obstruction.
Panic.
Scores of people will be quite upset when/if the details are released.
Fraud vitiates everything.
CodeMonkeyZ
The meeting was recorded?
There is a whistleblower?
> worriedface.jpg
What did they record?
Who is the whistleblower?
Who has the tapes?
Multiple copies?
Obstruction.
Panic.
CodeMonkeyZ
An emergency meeting has been called.
——
What does he know?
How does he know it?
Which meeting is he talking about?
Oh… That one.
What are the probabilities that there is a whistleblower?
What did we say in the meeting?
What is the potential blowback if the tapes are released?
How can we get ahead of this?
Proof of obstruction?
Who is the whistleblower?
PANIC.
Damage control.
CodeMonkeyZ
Chucky [Stephen Richer] won’t sleep well tonight.
—
Pawn.
Scapegoat.
In over his head.
Not privvy to the meeting?
Might take the fall for the meeting.
CodeMonkeyZ
Not everything I post is meant for the same audience.
Something that doesn’t make sense to you might be able to strike a specific chord in the person I am addressing.
Speaking of Blackrock.
On the way back from ATV’n in the desert, diverted through a new sub-division in the area.
These and ALL homes in the area are bought up as fast as they go on the market. Even before the slab is poured.
Most homes are occupied by folks with CA plates. A few Utah and Arizona. The rest NV.
Saw two homes with “for rent” signs. New homes. Never occupied.
Signs indicated, “Chase” and a phone number. Googled them.
One rental easily accepted as normal. Two on the same street, NAH. Something is up.
Will keep my eyes open.
Fascinating!
I have a theory.
Somebody thinks that the states are going to POLARIZE – and maybe even that is part of their strategy. They are predicting MASSIVE social movement when that happens. Many people will FLEE blue states, and others will FLEE red states. Urgency will DRIVE UP PRICES. Thus, buying home dwellings NOW will be a big moneymaker.
Taking a page out of NewScum’s commandeering/renting hotel space?
Need to have a place to put all those “migrants”…. on our dime, all the time…
Aztlan for rent…
Rent free… to the right (erm, left) community…
Different theory, although both would probably operate in the same direction, as blue states go nuts letting in foreigners.